Sintering entails heating and compacting a powdered material to create a solid mass with desirable properties. There are 13 different sintering methods, each with special benefits and uses. This includes pressure-assisted sintering, which improves densification, conventional sintering, a widely used technique, and the cutting-edge spark plasma sintering (SPS) technique, which is renowned for its quick and consistent results. The automotive, aerospace, and medical industries are just a few that sintering finds application. This article will further discuss all 13 techniques as well as the methods most commonly used in certain industries.
1. Conventional Sintering
In conventional sintering, also known as "free" or "pressureless" sintering, a powder compact is heated without the use of external pressure. The compact is formed by applying pressure, and it is subsequently expelled from a die cavity. Box furnaces can sinter larger materials like stainless steel, while tubular furnaces may sinter smaller materials like glass or ceramic powder. The heating elements in both types of furnaces are silicon molybdenum rods. Box furnaces provide more stable conditions whereas tubular furnaces circulate the air. In the practice of conventional sintering, a temperature of 1120 °C is employed for sintering over 80% of structural powder metal components. This approach encompasses both materials and methodologies that have yielded an economically viable means of shaping metals, endowing them with satisfactory mechanical attributes. For the mass manufacture of diverse parts, box furnaces have a wide range of uses in a variety of industries, including: agriculture, lawn and garden, and transportation
2. Pressure-Assisted Sintering
Pressure-assisted sintering, also known as hot pressing, synergizes elevated temperature and applied pressure to enhance material densification. Popular pressure-assisted processes include spark plasma sintering (SPS) and hot isostatic pressing (HIP). Reduced pore size and improved particle rearrangement are made possible by external pressure, which raises the mechanical characteristics. This method is very beneficial for materials that are difficult to densify naturally, like some ceramics. Additionally, pressure-assisted sintering's transformative effects have a significant positive impact on materials designed for high-density applications.
3. Spark Plasma Sintering (SPS)
With the help of the cutting-edge process known as spark plasma sintering (SPS), particles can be transformed into solid objects without melting them first. It applies pressure and heat quickly by using a high-density pulsed current and a regulated environment. With this innovative process, SPS may combine materials in a way that other techniques cannot, producing outcomes more quickly. SPS is adaptable and suited for a wide range of materials, including metals, ceramics, and nanomaterials. Advanced ceramics, thermoelectric semiconductors, intermetallics, and composites are a few examples of applications. SPS is popular in the aerospace, biomedical, and energy industries, and is advantageous for creating complex shapes.
4. Hot Isostatic Pressing (HIP)
Hot isostatic pressing (HIP) is a transformative manufacturing method that employs high pressure (ranging from 20 to 200 MPa) and elevated temperatures to create durable materials. The process capitalizes on packed powders that coalesce at temperatures exceeding half of their absolute melting point. Accurate atmospheric control is a key component of HIP. An inert environment, often using high-purity argon or nitrogen, is essential to protect the mechanical integrity and longevity of high-performance components. Due to full densification, which reduces internal porosity, HIP produces components that are almost net-shaped and have improved mechanical characteristics. This method has a wide range of applications, including eliminating residual porosity from sintered products, fixing casting faults, and powder pressure sintering.
5. Microwave Sintering
Microwave sintering employs electromagnetic waves to rapidly heat powdered materials, enabling quick and efficient sintering with the use of pressure. The process leverages the unique interaction between microwaves and polarized particles. This leads to internal heating and promotes the formation of homogeneous microstructures. This interaction accelerates diffusion rates, effectively reducing both processing time and energy consumption, making microwave sintering an environmentally friendly and cost-effective method. Ceramics, metal-ceramic composites, and ceramics derived from polymer precursors find applications in microwave sintering. The term "ceramics derived from polymers" refers to materials synthesized by converting polymer structures into ceramics through controlled thermal processes. An example is silicon carbide derived from polycarbosilane. Comparatively, spark plasma sintering (SPS) and microwave sintering share the goal of rapid consolidation, but they employ different mechanisms. SPS utilizes pulsed direct current and higher pressures, while microwave sintering employs electromagnetic waves and lower pressures, offering distinct routes to efficient material consolidation.
6. Reactive Sintering
Reactive sintering is a sintering process in which powders interact chemically, creating new phases and distinct microstructures. This method makes it possible to create advanced materials with specific properties, such as increased hardness and wear resistance. One significant application, for instance, is the creation of cemented carbides, which are widely used as cutting tools due to their excellent toughness and cutting capacity. The unique function of reactive sintering in the production of cutting tools involves the formation of carbides and other compounds, improving tool life and accuracy in machining operations.
7. Liquid-Phase Sintering
Liquid-phase sintering is a sintering process that uses a liquid phase to speed up the bonding of solid particles. Rapid particle rearrangement is first caused by capillary forces, and the process of densification is sped up by the liquid's improved diffusion. A mixture of powders, the melting of a single component, the formation of a eutectic, or the sintering of a material between the liquidus and solidus are all possible causes of the liquid phase. This method increases the toughness of normally brittle components by creating microstructures with hard particles inside of a ductile matrix. Cutting tool production and ceramic-matrix composites for aerospace are two businesses that benefit from liquid-phase sintering. Examples of products produced by this method include dental amalgam for fillings, electrical contact, oil-less bearings, high-temperature turbines, and soldering pastes.
8. Field-Assisted Sintering Techniques
Direct current (DC) or pulsed electric currents are used in field-assisted sintering techniques, such as spark pPlasma sintering (FAST/SPS), to heat molds and samples by joule. Compared to traditional sintering techniques, this leads to rapid heating rates and shorter processing durations. The advantages of FAST/SPS over conventional sintering, which involves heating powder materials to just below their melting temperatures, include the capacity to sinter a wide range of materials, including those with low melting points or prone to cracking. It ensures equal heat and pressure distribution while giving the sintering process fine control through the use of electric fields. With this method, materials with remarkable qualities might be produced with greater manufacturing efficiency and superior mechanical properties. It is used to create different metallic, ceramic, and functional materials.
9. Selective Laser Sintering (SLS)
Selective laser sintering is an additive manufacturing process that uses a high-intensity light beam to melt and bond successive layers of powdered materials to create three-dimensional objects. This method allows for a great deal of design flexibility. It is especially useful for rapid prototypes and production of parts with complex geometries in a variety of fields, including the automotive, aerospace, and medical device industries.
10. Freeze Sintering
Freeze sintering, also known as freeze casting, involves freezing a liquid suspension of powder particles and then sublimating a frozen solvent under reduced pressure. This leads to the consolidation and densification of the walls, resulting in a porous structure with unidirectional channels, resembling the solvent crystals. The technique exploits natural segregation phenomena, causing ceramic particles to accumulate between growing solvent crystals, analogous to salt, and biological entities in brine channels within sea ice. Sublimation of the solvent under low temperature and reduced pressure conditions creates porosity reflecting the solidified solvent structure. Finally, the green body is sintered conventionally to retain the macroporosity while removing microporosity from the ceramic walls. This unique process results in highly aligned structures with controlled porosity. Freeze sintering is beneficial for producing scaffolds in tissue engineering and ceramic materials with controlled porosity for thermal insulation applications.
11. Flash Sintering
Flash sintering is a rapid consolidation process for ceramics where high electric fields are applied during sintering, leading to densification at lower temperatures and shorter times compared to traditional methods like spark plasma sintering (SPS) or field-assisted sintering technology (FAST). Flash sintering utilizes intense electric fields to enhance ion transport and grain boundary diffusion, resulting in efficient densification. It finds applications in industries such as electronics, aerospace, and energy. Examples include: ceramic capacitors, thermoelectric materials, and solid oxide fuel cells.
12. Self-Propagating High-Temperature Synthesis (SHS)
Self-propagating high-temperature synthesis (SHS), also known as combustion synthesis, is an innovative technique where an exothermic reaction between powder components leads to rapid sintering. Initiated by an arc, a combustion wave propagates through the material, promoting fast sintering. High temperatures produced by this method induce sintering, which produces distinctive microstructures and characteristics. SHS has uses in vitrifying nuclear waste materials like Synroc, a second-generation waste form for the disposal of high-level radioactive waste. A study used SHS to effectively synthesize zirconolite (CaZrTi2O7), an important Synroc mineral, employing CuO and MoO3 as oxidants and Ti as a reductant. Variations in the TiO2/Ti ratios affected reactivity and adiabatic temperature, causing the synthesized samples to have the required density and Vickers hardness.
13. Induction Sintering
Induction sintering is a technique involving induction heating used for quick and precise sintering of powder compacts. While improving the mechanical properties of the material, this focused heating uses less energy. Due to its effectiveness and precise temperature control, induction sintering is advantageous for businesses that produce magnetic materials and metallic components. Two induction sintering methods are discussed: one uses a heated conductive container or die (susceptor), and the other induces eddy currents directly in the compact when it is placed inside a coil. These methods enable quick and effective sintering by shortening the processing time and assuring energy efficiency. Examples of successful induction sintering that preserve metastable phases include materials that are nanostructured and finely grained.
What Is Sintering?
Sintering involves heating powdered materials, such as metals or ceramics, slightly below their melting point to cause the particles to combine into a solid mass. Atomic diffusion across particle boundaries creates strong bonds to do this. Successful sintering decreases porosity and improves properties including strength, thermal conductivity, electrical conductivity, and translucency. Controlled sintering strengthens materials while maintaining porosity in some circumstances, as shown in filters and catalysts that need to be gas absorbent. Atomic diffusion removes surface flaws in the powder during the process, moving from pore formation to neck formation. The driving force behind the development of new, lower-energy solid-solid interfaces is the decline in free energy from surface reduction. By controlling the bond area, particle size, and vapor pressure, temperature, and grain size management have an impact on characteristics. The impact of temperature on diffusion and overall process quality is important. Figure 1 is a schematic diagram of the sintering process:
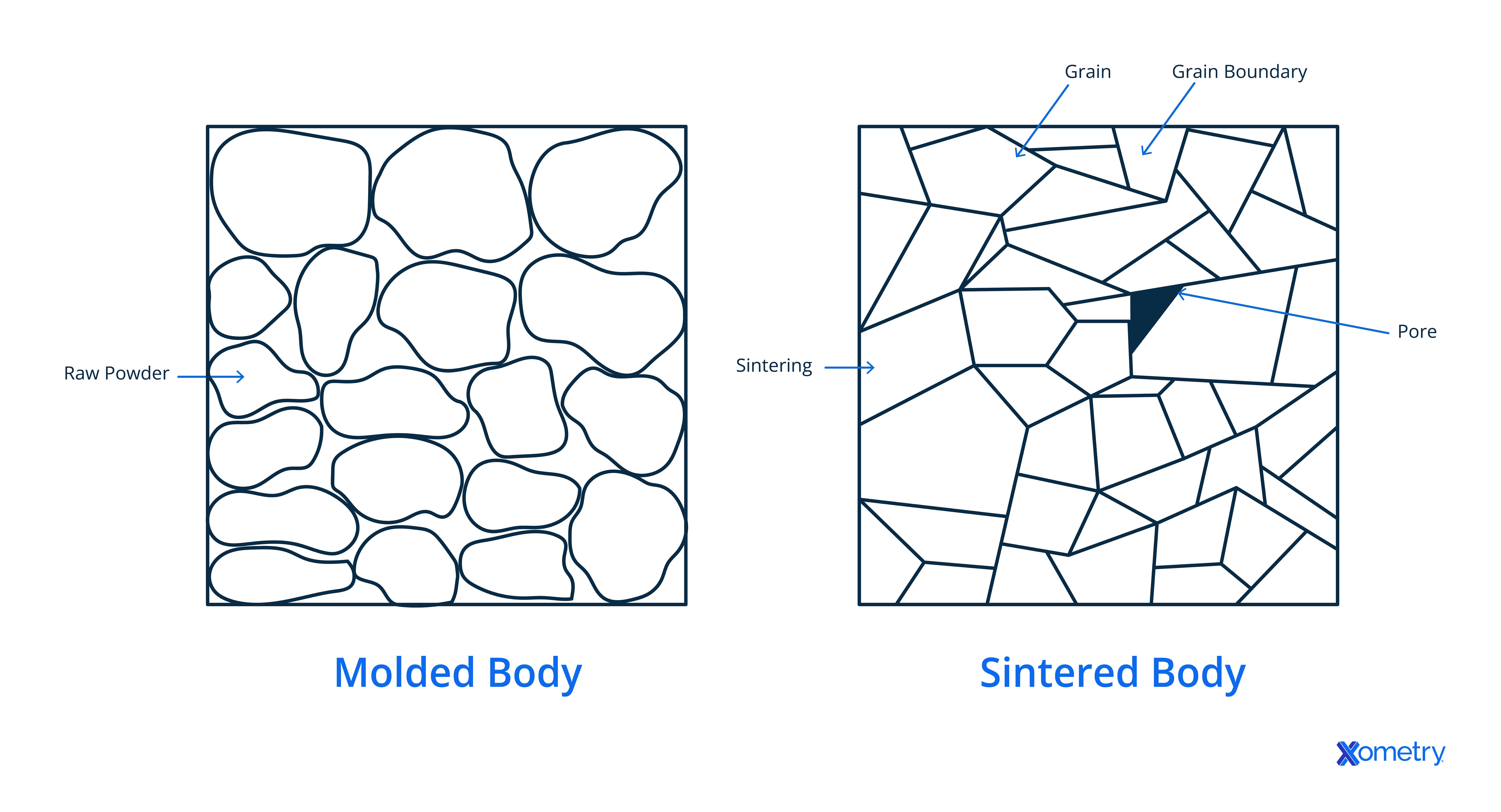
Sintering process schematic.
How To Choose the Best Type of Sintering?
To choose the best sintering method, it is important to consider the properties of the specified materials, desired product characteristics, and production requirements. Common techniques include conventional sintering, field-assisted sintering, and pressure assisted. Conventional sintering suits simple shapes, while fast-assisted sintering suits complex geometries and gives shorter processing times. Hot isostatic pressing is ideal for achieving high density and uniformity in critical components. Evaluate these factors to make an informed decision.
What Type of Sintering Is Used in the Automotive Industry?
Powder metallurgy and selective laser sintering (SLS) are two prominent sintering techniques utilized in the automotive industry. SLS uses a powerful laser to layer-by-layer fuse powdered plastic or metal elements, allowing the creation of complex components. This approach is preferred for quick prototyping, economical production, and design optimization. Powder metallurgy, on the other hand, uses metals that have been ground into a fine powder and then sinters them to produce complicated pieces. This method is essential in many automotive systems, including electronics, powertrain, and thermal management, because of its advantages in terms of precise tolerances, durability, and versatility.
What Type of Sintering Is Used in the Electronic Industry?
In the electronics sector, microwave sintering is a widely used procedure that uses microwave energy to stimulate the fusion of metals, composites, and ceramics. This methodology accelerates and improves sintering, outperforming traditional approaches like conventional sintering. It finds use in many different electronic parts and gadgets, and gains from faster processing times and better material qualities. Semiconductors, capacitors, resistors, and integrated circuits all fall under the category of electronic components and gadgets.
What Type of Sintering Is Used in the Aerospace Industry?
Pressure-assisted sintering (PAS) is one of the industrial processes used in the aerospace sector. It involves applying pressure to the green part while sintering. Compared to traditional sintering methods without pressure, PAS generally results in higher material density and lower porosity. High-performance aerospace parts, such as engine parts, structural components, and turbine blades, can all be manufactured using PAS.
Do Different Materials Require Different Sintering Processes?
Yes, different materials may require different sintering methods, though most methods can be used for an overlapping range of materials. The sintering process is an important step in the creation of materials, and different materials have different requirements and unique properties that influence the sintering method selection. These variations result from elements like melting points, electrical conductivity, and temperature sensitivity. For instance, the spark plasma sintering (SPS) technique works well when working with conductive materials like copper. Pulsed DC, used in SPS, enables quick and targeted heating while preserving the material's conductivity, preventing excessive grain formation. On the other hand, pressureless sintering, a traditional method that relies on diffusive mass movement during high-temperature sintering, is best suited for non-conductive materials like alumina. Also, materials with high melting points such as tungsten carbide necessitate specialized methods. Hot isostatic pressing (HIP) is particularly apt for such cases, as it combines high pressure and temperature in an inert gas environment to achieve high densities and eliminate porosities, thereby enhancing the material's strength and durability.
Do Sintering Types Differ in Desired Outcomes?
Yes, different sintering methods can be tailored to produce different desired results. Each sintering process has specific characteristics that influence the final properties and the cost of the sintered material. For example, microwave sintering can offer rapid heating, whereas pressure-assisted sintering can enhance material densification. The choice of method depends on factors such as part design, material choice, service conditions, and manufacturing efficiency.
How Does the Sintering Technique Affect Sintered Material Impact Test Results?
The results of impact tests can be greatly affected by the sintering process chosen. The density, porosity, and microstructure of the material can vary depending on the sintering technique. These factors directly affect the material's mechanical properties, such as toughness and strength. For instance, microwave sintering can lead to a more uniform microstructure and reduced porosity, enhancing impact resistance. A higher sintering temperature promotes diffusion bonding, making remaining pores more spherical and resulting in improved mechanical properties. Researchers found that with increased sintering temperature, components experienced a 30% rise in tensile strength, 15% in bending fatigue strength, and a remarkable 50% in impact energy, reflecting enhanced toughness. Impact strength notably rises with sintering temperature, with a more pronounced effect at higher carbon content due to increased retained austenite and bainite.
How Does Conventional and Pressure-Assisted Sintering Affect the Elastic Limit of Sintered Materials?
Conventional sintering may result in sintered materials with lower yield strengths, principally as a result of the persistence of porosity and flaws at grain boundaries. By increasing densification, lowering porosity, and fostering stronger grain boundary bonding, pressure-assisted sintering, in contrast, greatly improves the situation. Because of this, sintered materials have higher yield strengths. Applications that require enhanced mechanical performance, especially in terms of yield strength, benefit the most from pressure-assisted sintering.
Summary
This article presented the different types of sintering, explained each of them, and discussed when each are used. To learn more about sintering, contact a Xometry representative.
Xometry provides a wide range of manufacturing capabilities, including 3D printing and other value-added services for all of your prototyping and production needs. Visit our website to learn more or to request a free, no-obligation quote.
Disclaimer
The content appearing on this webpage is for informational purposes only. Xometry makes no representation or warranty of any kind, be it expressed or implied, as to the accuracy, completeness, or validity of the information. Any performance parameters, geometric tolerances, specific design features, quality and types of materials, or processes should not be inferred to represent what will be delivered by third-party suppliers or manufacturers through Xometry’s network. Buyers seeking quotes for parts are responsible for defining the specific requirements for those parts. Please refer to our terms and conditions for more information.
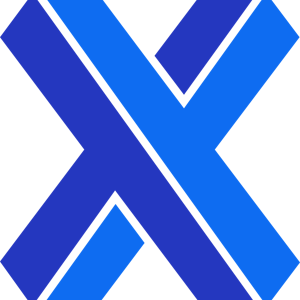