A laser diode (or diode laser) is a semiconductor device that undergoes stimulating emission to emit coherent light. Laser diodes offer high power for their size and produce electrical-power-efficient laser radiation. They consist of a p-n semiconductor junction, with a forward bias voltage applied to trigger a current through the junction. This induces population inversion (of electrons in the excited state) in the semiconductor material, biasing towards high-energy states. These results stimulate the emission of narrow-band coherent photons, frequency dependent on dopants in the semiconductor. Laser diodes are widely used across various industries, including: telecommunications, material processing, and medical treatments.
This article will discuss diode lasers, how they work, their applications, and their types.
What Is a Diode Laser?
Diode lasers are compact, solid-state devices that generate coherent light from semiconductor material. They are constructed using materials like gallium arsenide (GaAs) or gallium nitride (GaN). They operate by applying an electrical current to the semiconductor material, which stimulates the emission of coherent photons. Diode lasers are compact, making them ideal for portable applications. They can be designed to emit light across a wide range of wavelengths from ultraviolet (UV) to near-infrared (NIR) and mid-infrared (MIR). They can operate as continuous waves (CW) or pulsed emitters. Diode lasers are used in diverse sectors such as: telecommunications, data storage, barcode scanners, hair removal, medical/cosmetic treatments, displays, research tools, and manufacturing.
What Is the Origin of Diode Lasers?
The first diode lasers were developed in the early 1960s. The most significant steps were made by Robert N. Hall (General Electric, G.E.) who developed gallium arsenide (GaAs) IR laser diodes. Nick Holonyak Jr. (also G.E.) developed gallium arsenide phosphide (GaAsP) devices, emitting visible light, also in 1962. Zhores I. Alferov developed heterostructure lasers with multiple semiconductor junctions in the 1970s in the Soviet Union. This improved the efficiency and performance of diode lasers, making them more practical and usable.
How Does Diode Laser Work?
Diode lasers work by stimulating the emission of photons at a semiconductor junction. The semiconductor material has specific energy bandgaps that trigger the generation and amplification of coherent light. A diode consists of a p-n junction. The n-type region creates an excess of negatively charged carriers (electrons) while the p-type creates an excess of positively charged carriers (holes). The junction forms a depletion region between the two materials. When a forward bias voltage (+ve to the p and -ve to the n material) is applied across the junction, current flows. This causes charge carriers to move across the junction. Electrons from the n region and holes from the p region are injected into the depletion region. These meet and neutralize, releasing a photon for each charge canceled.
The diode laser is designed with reflective surfaces at the ends, forming an “optical cavity.” The photons reflect internally, and optical feedback enhances the stimulated emissions and results in narrow-band, coherent light. Stimulating emission also occurs when a photon interacts with an excited electron, causing it to emit another photon. These additional photons are identical to the triggering photon, leading to amplification. As the stimulated emission continues and photons are reflected within the cavity, the intensity of the laser energy increases.
What Is the Importance of a Diode Laser?
Diode lasers are valued because of the following:
- They're compact for their power levels. This makes them easy to integrate into smaller systems.
- Applications benefit from higher power efficiency than alternative laser types, as they convert electricity into laser power with low wastage.
- Design choices offer wavelength flexibility. Diode lasers can emit light from the ultraviolet (UV), through visible to near-infrared (NIR) regions. Wavelength selectability makes the class ideal for many applications.
- They offer high-speed operation and precise control over the laser power. Rapid response and precise beam focusing enable highly effective energy for cutting, engraving, and drilling.
- Diode lasers achieve long and reliable operational life. This makes them suitable for long continuous operation.
- The accelerating development has resulted in improving power output, efficiency, beam quality, and reliability.
What Is the Primary Use of Diode Lasers?
Primary uses vary across a wide spectrum of applications/fields, including:
- Infrared laser diodes are key to fiber-optic data transmission. The short wavelength enables higher packet rates and frequencies, which in turn produces high data density.
- Laser diodes are key to many printing applications, with high beam quality allowing very high resolution of the printed image.
- They are used for many medical and cosmetic procedures such as hair removal, skin growth stimulus, tattoo ablation, vascular lesion treatments, and dental procedures. Controlled application to target tissues minimizes damage to the surrounding areas.
- They are used across manufacturing for cutting, welding, marking, engraving, and surface treatment. High-speed, precision processing makes them valuable tools.
- Diode lasers are commonly applied in scientific research, in fields such as spectroscopy and microscopy. They are useful in the analysis of molecular structures, chemical compositions, and biological materials.
- They are widely used in consumer devices such as laser pointers, barcode scanners, DVD and Blu-ray players, and optical data drives. Compactness and low-power demand are key to these applications.
- They are key to various sensing applications such as distance measurement, range finding, and lidar systems. They are used in metrology tools for precise measurements in industrial and scientific applications such as 3D scanning, dimensional inspection, alignment, and calibration.
What Are the Different Types of Diode Laser?
There are several types of diode lasers, each suited to specific application classes. These are listed below:
- Edge-emitting diode lasers, or Fabry-Perot lasers, are the most common. They emit laser light perpendicular to the junction plane.
- Vertical-cavity surface-emitting lasers (VCSELs) emit laser light perpendicular to the wafer surface. They employ a distributed Bragg reflector (DBR) for high reflectivity and efficient emission.
- The quantum cascade laser (QCL) is a specialized type of diode laser that operates based on intersubband transitions, in quantum wells. They can emit laser light across the mid-infrared (MIR) and far-infrared (FIR) regions by adjusting the forward bias.
- Distributed feedback (DFB) lasers are edge-emitting diode devices with distributed feedback gratings. The grating enables wavelength selectivity, resulting in a narrow line, single longitudinal mode emission.
- External cavity diode lasers (ECDLs) use an external cavity with a diode laser chip to provide tunable and narrow linewidth laser output. They are widely used in spectroscopy, metrology, and nuclear physics research.
- Superluminescent diode (SLD) lasers were developed for broad-spectrum, low-coherence light output. They are used in applications in which broadband and low-coherence light sources are appropriate.
What Are the Main Applications of Diode Lasers?
Diode lasers have many applications, including those listed below:
1. Medical
Diode lasers perform a wide range of roles related to medical services, resulting from their compactness, durability, and flexibility. These lasers are used in various medical applications including: hair removal, skin treatments, soft tissue surgery, photodynamic therapy (PDT), endovenous laser treatment (EVLT) of varicose veins, and low-level laser therapy (LLLT). For example, for LLLT, diode lasers are employed for pain management and tissue healing. The laser penetrates overlying tissue, stimulating cellular metabolism, reducing inflammation, and alleviating pain.
2. Printing
Laser diodes have various printing and print-related applications across many sectors. Laser diodes are at the heart of laser printers. They are the light sources for the printing process; the beam scans across a photoreceptive surface to create an electrostatic image for toner attraction. They are also used in barcode and QR code printers, locally heating thermally sensitive paper to apply the barcode or QR codes. Additional uses of diode lasers are employed in: selective laser sintering (SLS) or selective laser melting (SLM) to build 3D models, laser engraving and marking systems to etch a range of materials, and printing banknotes, passports, and official documents, to embed security features like holograms, micro text, or covert markings.
3. Telecommunications
Diode lasers are used in fiber-optic communication systems. They are the light source for transmitting data. In long-distance fiber-optic links, signal amplification is necessary to overcome signal degradation. Erbium-doped fiber amplifiers (EDFAs) are used for this purpose. In optical time-domain reflectometry (OTDR)—a fiber-optic testing method—a laser emits short pulses of light into the fiber, and the reflected (backscattered) light is analyzed to determine fiber loss and locate fiber breaks or kinks. Additional uses of diode lasers are found in the transmission of data through the air, and in wavelength division multiplexing (WDM) to increase the capacity of optical communication systems by transmitting multiple signals simultaneously, at offset wavelengths.
4. Spectroscopy
Laser diodes are very well adapted to use in spectroscopy, enabling precise and sensitive analysis of materials and compounds. Raman spectroscopy involves shining laser light onto a sample, so the backscattered scattered light is analyzed to obtain information about the constituents and structural characteristics of the material. Laser diodes can be tuned to the Raman shift of interest, allowing for selective excitation and detection. Laser diodes are also used as the excitation sources in fluorescence spectroscopy, which illuminates a sample so the emitted fluorescence can be measured to identify substances. Laser diodes deliver virtually monochromatic light, which allows for precise excitation. Additional applications are: diode laser absorption spectroscopy (DLAS) or tunable diode laser absorption spectroscopy (TDLAS), cavity ring-down spectroscopy (CRDS), laser-induced breakdown spectroscopy (LIBS), and laser-induced fluorescence (LIF) spectroscopy.
5. Sensing
Laser diodes are widely used in sensing applications because coherent light allows easy observation of changes in the reflected or transmitted light frequency or phase of the target. Laser diodes are utilized in the distance and position measurement. Laser triangulation sensors project a laser beam onto a target to determine the distance or position. These sensors are used in robotics, automation, and metrology. Other applications include: light detection and ranging (LiDAR) systems, laser doppler velocimetry (LDV) systems, and flow and level sensing.
6. Materials Processing
Laser diodes are widely used in materials processing applications due to their compact size, high power, and electrical efficiency. Laser diodes are increasingly used in laser cutting systems for the automated cutting of a range of materials. Laser diodes provide a tightly focused beam that gives high energy density. This allows accurate and rapid cutting of various materials. They are also common in welding applications, in which the focused beam fuses materials by melting/merging. Laser welding is increasingly important in the automotive, jewelry, and electronics sectors.
Drilling and micromachining use the precisely focused beam of laser diodes to create small diameter holes in metals, ceramics, and semiconductors. Laser micromachining serves to remove and shape small cuts/ablations with high precision for the manufacturing of microelectromechanical systems (MEMS), etc.
How To Use Diode Laser?
Laser diodes have diverse applications and usage scenarios. In general, laser diodes are integrated into functional equipment for a variety of applications, including:
- Handheld medical lasers for skin ablation, self-cauterizing cutting, hair follicle destruction, vein closure, and a multitude of imaging tools.
- Industrial processing tools for cutting, welding, cleaning, ablating, and drilling various materials.
- As the data transmitter in fiber-optic systems.
- Long-distance direct line of sight “tight beam” data transmission.
- Measurement of distance, speed, material type, and other properties.
How Is the Wavelength of a Diode Laser Determined?
The wavelength of a diode laser results from the properties of the semiconductor material used in the device. The bandgap energy controls this outcome and it is highly tunable. Some examples are listed below:
- Semiconductor material selection plays the most important role in determining the wavelength. Varying bandgap energies correspond to wavelengths of light. Gallium arsenide (GaAs) is commonly used for infrared diode lasers, while gallium nitride (GaN) is used for blue or ultraviolet devices.
- The bandgap wavelength relationship defines the minimum energy required for an electron to transition between the valence and conduction bands. Each electron transition releases a photon of a particular wavelength. This relationship between bandgap energy (Eg) and wavelength (λ) is defined by the equation λ = c / Eg, where c is the speed of light in a vacuum.
- Quantum well or quantum dot structures confine electrons and holes to a narrow region, modifying the bandgap structure, and narrowing the emitted spectrum.
- Temperature dependence influences the bandgap energy. This causes a change in the emitted wavelength.
What Is the Typical Output Power of a Diode Laser?
There is no “typical” output power for a laser diode, as energy levels vary significantly. The output power of a laser depends on the design of the laser, the properties of the semiconductor materials, and the cooling employed. Power levels vary from milliwatts (or even microwatts) to tens of kilowatts. For example: low-power diode lasers (up to tens of milliwatts) are used in applications such as laser pointers, barcode scanners, CD/DVD drives, and biomedical sensing. Medium power devices (10 mW to 30 W) are used in a variety of applications such as laser printing, projection systems, and fiber optics. Higher-power devices (30 W to 10+ kW) are used in industrial, scientific, and medical applications that require these higher power levels.
What Are the Best Practices for Aligning a Diode Laser?
Aligning a diode laser requires care to ensure efficient operation. Some best practices are listed below:
- Mounting and stability—both systems and the laser device within them—greatly affect performance in most laser-based systems.
- Begin by roughly aligning the diode laser and the optical components along the desired optical path. Use alignment tools such as: beam dumps, beam profilers, or beam expanders.
- Perform coarse alignment by adjusting the position and angle of the laser source and optical components. Follow the alignment procedure recommended by the equipment supplier/maker.
- Beam manipulation tools, such as beam profilers or beam viewers allow you to observe and analyze the beam profile, enabling optimization of the alignment.
- Power monitoring with a photodiode monitors the output power of the laser, allowing you to assess the effect of alignment adjustments on the beam quality.
- Small iterative adjustments enable fine-tuning. Perform microchanges to the position, tilt, and rotation of the optical components while monitoring the beam profile and power output.
Thermal management is important, as diode lasers are sensitive to temperature changes. Ensure proper cooling of the laser system.
How Can Diode Lasers Be Applied in Laser Welding?
Diode lasers are widely used in laser welding applications due to their high power, compact size, durability, and efficiency. They offer various advantages for laser welding, including: high power efficiency, compact size, excellent control/repeatability, and ease of integration into production processes. Select the device based on output power, beam quality, wavelength, and pulse duration to suit the requirements. The beam delivery system guides the laser beam from the diode source to the weld. This consists of focusing and beam-shaping optics and protective covers. Focusing the beam delivers the required spot size and focal point position relative to the workpiece. This delivers the necessary energy intensity. The parameters can be adjusted to optimize power, pulse duration, pulse rate, shield gas flow, and feed speed. Optimizing these for joint design, material type, and material thickness is critical for weld quality.
Begin the welding process by initiating a melt-fusion zone. Then start feeding along the joint line to maintain the melt-fusion pool. A consistent gap and alignment between the workpieces ensures uniform and sufficient/consistent penetration.
How Are Diode Lasers Used in Laser Cutting?
Diode lasers are also widely used in laser cutting applications, due to their high power, compact size, and power efficiency. Choose a diode laser suitable for laser cutting applications. This considers factors such as output power, beam quality, wavelength, and pulse duration to suit the cutting process and the materials to be cut. Prepare the workpiece to be cut by cleaning and positioning it in the desired configuration. Beam delivery guides the energy from the source to the cutting area. This requires focusing and beam-shaping optics, plus protection to exclude or minimize debris getting into the optical path.
Focusing the beam to achieve the required/recommended spot and kerf properties will improve the cut rate and quality and minimize the heat-affected zone. Cutting parameters such as beam power, pulse duration, pulse rate, and feed speed depending on the material/thickness being cut and the desired cut quality. These parameters are adjusted to achieve a clean cut, minimal HAZ, and high throughput. For more information, see our guide on what is a laser cutter.
Do UV Diode Laser Used in Laser Cutting?
No, UV diode lasers are not generally used for laser cutting applications. These lasers, however, offer shorter wavelengths which are beneficial for some applications such as precise ablation or surface modification. In particular, the typical material absorption of UV light by most materials results in surface ablation or modification and a wide HAZ, rather than efficient cutting. While UV lasers are extensively used in micromachining, lithography, semiconductor processing, and medical procedures they are not suited to fine cutting.
How Much Does Diode Laser Machine Cost?
The price of laser diode machines hinges on power requirements, required frequency of emission, and optical quality issues. Compact diode laser systems with power output up to 30 W can cost from a few dollars to tens of thousands of dollars, depending upon quality and application issues. For high-power devices used in industrial applications such as commercial laser cutting, welding, or surface treatment, the cost can range from tens of thousands of dollars to hundreds of thousands of dollars and more.
It should be noted that the cost of a diode laser machine is only partially determined by the cost of the laser itself. The rest of the machine will be the largest part of the cost.
What Is the Life Span of a Diode Laser Machine?
The life span of a diode laser is influenced by the quality of the diode materials, manufacturing processes, intensity of use, overheating influences, and operating conditions. Diode lasers typically have a life expectancy ranging from thousands to tens of thousands of hours.
What Are the Safety Considerations When Using Diode Lasers?
When using diode-laser-based equipment, accepted basic safety precautions must be adhered to, including:
- Understand the safety measures and precautions associated with the laser classification of your equipment.
- Use the right laser safety goggles or eyewear for the laser wavelength of your equipment. Ensure that anyone operating or in the vicinity is using appropriate PPE.
- Diode-laser-equipped machines must have interlock mechanisms to prevent accidental exposure to the beam.
- Display appropriate laser safety signage for the class of equipment.
- High-power devices (typically 1 kW and above) require a controlled area. Restrict access.
- Provide appropriate operational safety training for staff working with or around the machine.
- Make sure that the beam is enclosed, to prevent exposure. Use beam blocks or beam dumps to terminate the beam without flash or reflection.
- Be aware of potential fire hazards, as with any hot process. Ensure fire extinguishers are on hand.
- Regularly inspect and maintain the equipment and environs, to manage risks.
- Set up emergency procedures in case of accidents. This includes protocols for laser injuries, reporting incidents, and seeking medical attention.
How Does Temperature Affect the Performance of a Diode Laser?
Temperature can significantly affect the performance of a diode laser. Output power and efficiency are affected by temperature. As temperature rises, the diode's electrical resistance decreases, leading to an increased current and output power. Wavelength instability can result from overheating. Higher-precision devices incorporate thermoelectric (Peltier) coolers, to improve stability. Temperature can also degrade beam quality by increasing beam divergence and altering the beam profile. This is a result of refractive index changes within the semiconductors.
What Are the Advantages of Diode Lasers?
Diode lasers integrate a range of advantages over other laser systems that make them a good choice in many applications. These are listed below:
- Their excellent power-to-weight/size ratio and limited need for ancillary equipment make them easy to integrate into equipment.
- Diode lasers' high electrical power efficiency means less power used per watt of laser energy emitted.
- They are electrically pumped, not requiring additional optics and external laser pumps.
- They can operate in continuous wave (CW) or pulsed mode, increasing their application flexibility.
- These devices can be constructed in a wide range of emission wavelengths, from ultraviolet (UV) to infrared (IR).
- Diode lasers offer close control of the laser output in intensity, frequency, and pulse width modulation.
- They generally have much longer operational lifetimes and require less maintenance than most alternatives, when correctly operated.
- They are fast to start and shut down, allowing for greater system availability.
- They are a class of devices with wide-ranging power outputs, allowing precise specifications for the application.
What Are the Disadvantages of Diode Lasers?
Laser diodes also have some severe disadvantages, including:
- Multi-mode beam quality is typically low when compared with solid-state or gas lasers. Single-mode devices offer better beam quality.
- Ultraviolet (UV) and deep infrared (IR) diode lasers can be difficult and expensive to make.
- Diode lasers are very sensitive to temperature fluctuations. Effective thermal management and temperature stabilization systems are required for stability.
- Diode lasers typically have lower power capabilities than fiber or CO2 lasers.
- Lifetime and reliability are shortened by bad operating conditions.
- Diode lasers are relatively expensive, in comparison with other systems.
- Beam divergence is greater than some comparable devices/systems, limiting the focusing ability.
Is Diode Laser a Semiconductor Lasers?
Yes, diode lasers are a type of semiconductor laser. Semiconductor lasers exploit the principle of “stimulated emission” of photons in the semiconductor material.
What Is the Difference Between a Diode Laser and Other Types of Lasers?
Diode lasers are unique in most regards, particularly in their operating principles, construction, and operational properties. These differences include:
- The operational principle is population inversion within a solid semiconductor, at a p-n junction. Gas lasers rely on population inversion in a gas.
- They are unique in directly generating the laser photon stream in a semiconducting medium.
- Most other laser devices require an optical pumping system from discharge, flash, or a low-power laser. Diode lasers use pure electrical pumping to initiate the light.
- Diode lasers offer the smallest package size per watt of the output of any laser system.
- The electrical pumping and minimal need for cooling, generally result in higher electrical efficiency than any other laser system.
- Diode lasers can be tuned to a wide spectrum of wavelengths and, in some cases, sweep a spectrum. Other laser systems emit more fixed wavelengths.
- They cannot (yet) achieve the extreme power of some other systems, although the pace of development remains rapid.
What Is the Difference Between a Single-Mode and Multi-Mode Diode Laser?
Single-mode and multi-mode generally describe the different propagation modes of light within an optical fiber, waveguide, or diode laser. Some differences are listed below:
- Single-mode diode lasers emit light in a well-defined and tightly confined path with a small diameter and low divergence, resulting in a narrow and focused beam. Multi-mode diode lasers emit light in multiple spatial modes with a larger beam diameter and higher divergence.
- Single-mode diode lasers provide better beam quality than multi-mode devices. The single-mode beam has a more uniform intensity profile, lower divergence, and better spatial coherence. This makes single-mode diode lasers suited to precise focusing, high spatial resolution, and longer propagation distances.
- Single-mode diode lasers are typically used with single-mode optical fibers, which have a small core size (typically around 9–10 micrometers). Multi-mode devices are typically connected to multi-mode fibers, which have a larger core size (e.g., 50 or 62.5 micrometers) to accommodate the wider beam.
- Single-mode diode lasers are better suited for high-precision applications and long-distance communication, suffering less dispersion and attenuation. Multi-mode diode lasers suffer from higher dispersion and attenuation effects due to the broader beam.
- Single-mode diode lasers and associated fiber components tend to be more expensive than multi-mode counterparts, due to the need for greater precision in manufacture.
What Is the Difference Between a Diode-Pumped Solid-State Laser and a Diode Laser?
A diode-pumped solid-state laser uses diode lasers as the pump source, and then a solid-state laser as the amplification device that generates the final beam. A diode laser produces the semiconductor laser light directly, not requiring an intermediate solid-state laser stage. Most laser types require an optical energy source to initiate the laser beam, whereas diode lasers use direct electrical current as the initiator of the population inversion that generates laser light.
Summary
This article presented diode lasers, explained them, and discussed their various applications. To learn more about diode lasers, contact a Xometry representative.
Xometry provides a wide range of manufacturing capabilities, including laser cutting and other value-added services for all of your prototyping and production needs. Visit our website to learn more or to request a free, no-obligation quote.
Disclaimer
The content appearing on this webpage is for informational purposes only. Xometry makes no representation or warranty of any kind, be it expressed or implied, as to the accuracy, completeness, or validity of the information. Any performance parameters, geometric tolerances, specific design features, quality and types of materials, or processes should not be inferred to represent what will be delivered by third-party suppliers or manufacturers through Xometry’s network. Buyers seeking quotes for parts are responsible for defining the specific requirements for those parts. Please refer to our terms and conditions for more information.
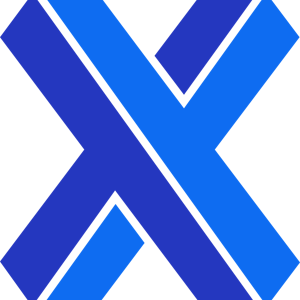