The iron-carbon phase diagram is a graphical representation of the different phase states that iron and its alloy can experience. It is a great tool for understanding the microstructure of iron and iron alloys and how they should behave. This article will help you understand what the diagram is, how it works, and the information it contains on the different states of iron and iron alloys.
What Is the Iron Carbon Phase Diagram?
The iron-carbon phase diagram is a chart representing the microstructure of the iron or steel caused by the temperature treatment and carbon content of the iron or iron alloy. All alloys have different phase states which are determined by their precise chemical composition and the arrangement of the bonds between these elements. The carbon content of iron alloys, such as steel, has a great effect on the microstructure of steel. However, if the steel receives rapid heating or cooling, or the other alloying elements are increased, then the smaller the importance of the carbon content. Figure 1 below is an iron-carbon phase diagram, with the applicable temperature during heat treatment on the Y axis and the carbon content on the X axis:
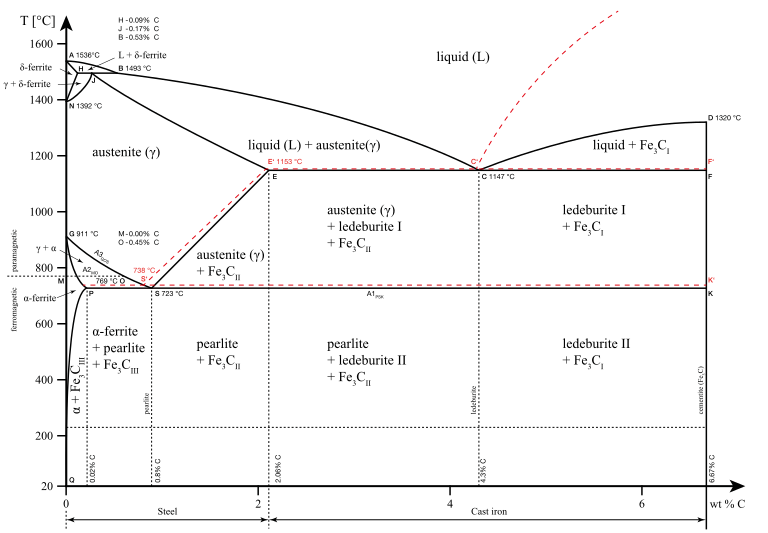
The iron carbon phase diagram.
Image Credit: https://en.m.wikipedia.org/wiki/File:Iron_carbon_phase_diagram.svg
What Is the Other Term for Iron Carbon Phase Diagram?
There are several other names that might be used to refer to an iron-carbon phase diagram such as: metastable iron-carbon phase diagram, Fe-C phase diagram, iron-iron carbide phase diagram, and Fe-Fe3C phase diagram. A metastable iron-carbon phase diagram is used to emphasize the fact that the iron-carbon relationship is metastable due to the slow cooling rates of iron alloys. In the term “Fe-C phase diagram,” Fe-C is shorthand for iron-carbon. The diagram is also known as a “steel phase diagram” since iron alloys are steel. “Steel” is used in place of iron-carbon to show that the diagram is used to understand its microstructure. “Iron-iron carbide phase diagram” is used to represent the iron carbide part of the phase diagram. In the term “Fe-Fe3C phase diagram,” Fe-Fe3c is shorthand for iron-iron carbide.
What Is the Origin of the Iron Carbon Phase Diagram?
The iron-carbon phase diagram was developed to show the relationship between carbon content and temperature treatment. The diagram was not invented in one single instance but was the result of a series of works coming together to form what is now considered the iron-carbon phase diagram. In 1897, Sir William Chandler Roberts-Austen used information gathered by an Adolf Martens microscope, which could study the grain structure of the metal and data from a device he invented to measure the temperature of melted iron to plot a T-x diagram. The T-x diagram isn't a true iron-carbon diagram because it is not in equilibrium. However, it is considered to be the basis of an iron-carbon diagram.
How Does an Iron Carbon Phase Diagram Work?
For an iron-carbon phase diagram, the temperature is plotted on the Y axis and carbon content, as a percentage of the weight, is plotted on the X axis. The graph is split up into segments, and each segment represents a different phase of the microstructure. The microstructure is therefore a function of the temperature and chemical composition of the iron. This means that, although two iron-carbon alloys can have the same chemical composition, if they are exposed to a different temperature, they will have different microstructures.
What Is the Importance of the Iron Carbon Phase Diagram?
The iron-carbon phase diagram is an important tool used to understand the microstructure of iron-carbon alloys. It is important to use a graph to demonstrate how the function of temperature and carbon content affect iron-carbon alloys, as without this it would be hard to communicate at what temperature and carbon content austenite becomes austenite + cementite (for example).
What Are the Different Phases in the Iron Carbon Phase Diagram?
The different phases in the iron carbon phase diagram are listed and discussed below:
1. δ-ferrite
ẟ-ferrite is a low-carbon (almost pure iron) phase that has a body-centered cubic crystal structure. With an increase in carbon content, cementite will begin to form creating ferrite + cementite. δ-ferrite is stable up to 912 ºC. Above 912 ºC, δ-ferrite transforms into a face-centered cubic austenite. δ-ferrite is very magnetic and ductile but has a low strength.
2. γ-austenite
y-austenite is a solid face-centered cubic phase, which is stable up until 1,395 ºC, at which point it transforms into a body-centered cubic ferrite. Most iron alloys that receive heat treatment start in the γ-austenite phase. y-austenite is non-magnetic, soft, and ductile.
3. α-ferrite
α-ferrite is a high-temperature iron-carbon phase that is created by cooling a low-carbon concentration in the liquid state. The liquid state is then cooled to an austenite phase. The presence of α-ferrite in iron-carbon resists lattice dislocation and therefore slows grain growth, reduces vulnerability to fatigue, and increases strength.
4. Fe3C (Cementite)
Fe3C is the chemical name for cementite, also known as iron carbide, which starts to be present at any carbon content above zero, but no iron is fully cementite until it includes 7% carbon content. Cementite can be produced through the cooling of austenite or the tempering of martensite. Cementite is hard, but also brittle, but cementite’s best property is its corrosion resistance which can be achieved when dipping cementite in a solution of 1–3% sodium chloride.
5. Ledeburite
Ledeburite is a mix of austenite and cementite and has a carbon content of 4.3% carbon. The carbon content of ledeburite is too high to be found in steel but is found in cast iron. Ledeburite has a melting point of 1,147 ºC.
6. Pearlite
Pearlite is formed when an austenitic phase of an iron-carbon alloy is cooled slowly. Pearlite is formed of alternating layers of ferrite and cementite, which gives pearlite significant toughness and strength.
7. Martensite
Martensite is created by rapidly cooling a face-centered cubic austenite phase. This process is referred to as a martensitic transformation. The martensitic transformation creates great hardness and strength. Quenching is used to invoke a martensitic transformation.
What Is the Purpose of Heat Treatment in the Context of the Iron-Carbon Phase Diagram?
The purpose of heat-treating iron-carbon alloys is to change the microstructure of the alloy and therefore its properties. By changing the rate of cooling, the properties of an iron alloy can be altered. When cooling pearlite at a rate of 200 ºC per minute, a hardness of 300 DPH can be achieved. Cooling at 400 ºC can achieve a hardness of 400 DPH. The reason there is an increase in hardness is due to the formation of fine pearlite and ferrite microstructure in the pearlite. This is because carbon has less time to move through the lattice structure of the pearlite as it cools. Therefore, when cooling, using liquid quenching at 1,000 ºC, the carbon has no time to move. This forms a martensitic microstructure that has a hardness of 1,000 DPH. Martensite has the hardest and most brittle microstructure of all iron alloys, but after tempering by holding martensite at 400 ºC it becomes less hard and brittle.
What Is the Most Important Temperature in the Iron-Carbon Phase Diagram?
There are many important temperatures in the iron-carbon phase diagram. One of the most important is 723 ºC, which is known as the critical or A1 temperature. It is the point at which any austenite in iron, cast iron, or an iron alloy transforms into the eutectoid pearlite. At this point, the microstructure has double the amount of ferrite as it does pearlite.
What Are the Different Reactions in the Iron Carbon Phase Diagram?
The two factors that affect the microstructure of iron-carbon alloys are temperature and carbon content. For a given carbon content, the heat treatment will affect the structure of the iron-carbon mix by changing the bonds between the elements. There are three types of bonding which are discussed below:
1. Eutectoid
A eutectoid reaction occurs at the eutectoid point at which one single solid phase transforms into two solid phases simultaneously upon cooling. An example of the eutectoid point on the iron-carbon phase diagram is at 0.8 %wt and 723 ºC. At this point, during cooling, austenite is transformed into ferrite and cementite.
2. Eutectic
A eutectic reaction is very similar to an eutectoid transformation. However, an eutectic reaction starts with a liquid and ends up as two solids, whereas a eutectoid starts as a single solid phase. The eutectic reaction will occur at the melting point of the mixture as it cools, and so the specific temperature will depend on the composition of the mixture.
3. Peritectic
A peritectic reaction occurs when a molten phase and a solid phase react to form a secondary solid phase. Peritectic reactions are not as common as eutectoid or eutectic reactions and there is only one form of peritectic reaction in the iron-carbon system.
How Can the Iron-Carbon Phase Diagram Help Engineers and Metallurgists?
The iron-carbon phase diagram can be used to predict the phase states within the material if the heat treatment it has received and the chemical composition is known. Since the physical properties of an iron-carbon alloy depend on the phases of the iron-carbon alloy, the iron-carbon phase diagram can aid engineers and metallurgists in predicting the properties and behavior of such an alloy. For example, if an iron-carbon alloy of 0.8% carbon is heated above 723 ºC and then liquid quenched, then it is known that martensite is formed and the iron-carbon alloy will be hard but brittle.
How Does the Quenching Rate Affect Steel Alloy Microstructure?
The aim of quenching is to alter the microstructure of iron-carbon alloys to increase their hardness. This works because when iron carbon is heated above a certain temperature, it transforms into an austenite phase which has a face-centered cubic structure, which can easily dissolve carbon atoms. Iron carbon which cools slowly at 400 °C per minute, for example, allows the dissolved carbon atoms to be released from the lattice structure. However, when austenite is quenched, at 1,000 ºC per minute, the carbon becomes trapped in the lattice. This increases the metal's internal stresses and makes the metal hard and brittle.
To learn more, see our guide on Steel Alloy.
How Does the Iron-Carbon Phase Diagram Help Predict the Mechanical Properties?
The iron-carbon phase diagram maps out all of the possible temperature treatments and carbon contents an iron-carbon alloy can experience. If the temperature and carbon content of an iron alloy are known, then the iron-carbon phase diagram can be used to predict in which phase states the iron-carbon alloy now exists. Iron-carbon alloys are well known and tested, so if the phase state of the iron is known, the mechanical properties can be predicted from known data. For example, martensite is known for being the hardest and most brittle steel. It can be predicted that if steel has been heated to above 723 °C and then quenched, it will exist in a martensitic state.
What Are the Types of Steel Used in the Iron Carbon Phase Diagram?
The types of steel used in the iron-carbon phase diagram are discussed below:
1. High-Carbon Steel
High-carbon steel, also known as carbon tool steel, has a carbon content of 0.60–1.50%. The high carbon content means it is harder and stronger than lower-carbon steels but it is also less ductile. High-carbon steel is also more corrosion-resistant than lower-carbon steel, if there is no addition of other alloying elements, such as chromium which can affect the corrosion resistance of steel. High-carbon steel is used in cutting tools, dies, springs, and high-strength wire.
2. Medium-Carbon-Steel
Medium-carbon steel has a carbon content of between 0.30–0.60%. Medium-carbon steel is the range that has the best balance between the properties of low- and high-carbon steel. Although medium-carbon steel is harder and stronger than its low-carbon counterparts, it often requires quenching to achieve the desired hardness. Medium-carbon steels are commonly used in: pressure vessels, gears, shafts, axles, and machinery parts.
3. Low-Carbon Steel (or Mild Steel)
Low-carbon steel has a carbon content between 0.05–0.30%. Low-carbon steels are known for their ductility and malleability. Due to the small amount of alloying carbon, low-carbon steel is the cheapest and most widely used as a general-purpose steel. Low-carbon steel is used for: fasteners, building frames, bridges, body panels, and pipework.
What Are the Advantages of the Iron-Carbon Phase Diagram?
Iron carbon diagrams are in widespread use due to their advantages which include:
- It is relatively easy to interpret.
- It can display information on a large number of phases.
- It is accurate.
- The information is well supported by research.
What Are the Disadvantages of the Iron Carbon Phase Diagram?
There are also disadvantages to the iron-carbon phase diagram which are:
- The diagram does not include all information, such as the non-equilibrium martensite phase.
- There is no time indication for rates of heating and or cooling.
- There is no indication of the exact properties of the different phases.
Is the Iron Carbon Phase Diagram Accurate?
Yes, generally iron-carbon phase diagrams are accurate. However, the accuracy of the diagram will vary depending on the origin of each diagram. There are some limitations of the diagram, such as the omission of bainite and martensite metastable phases. It also does not give time-dependent information on the formation of pearlite, bainite, or spheroidite.
Summary
This article presented the iron carbon phase diagram, explained it, and discussed how it works To learn more about iron carbon phase diagram, contact a Xometry representative.
Xometry provides a wide range of manufacturing capabilities and other value-added services for all of your prototyping and production needs. Visit our website to learn more or to request a free, no-obligation quote.
Disclaimer
The content appearing on this webpage is for informational purposes only. Xometry makes no representation or warranty of any kind, be it expressed or implied, as to the accuracy, completeness, or validity of the information. Any performance parameters, geometric tolerances, specific design features, quality and types of materials, or processes should not be inferred to represent what will be delivered by third-party suppliers or manufacturers through Xometry’s network. Buyers seeking quotes for parts are responsible for defining the specific requirements for those parts. Please refer to our terms and conditions for more information.
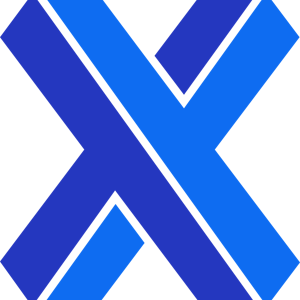