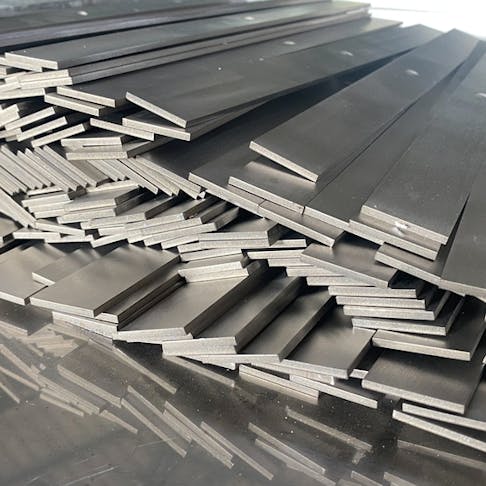
Precipitation Hardening Stainless Steel: What It Is, How It Works, Process, and Advantages
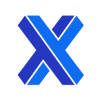
Precipitation hardening stainless steel creates a remarkable class of alloys that combines the advantageous properties of both martensitic and austenitic grades. These alloys undergo a specialized heat treatment process that significantly enhances their strength while preserving workability and ductility. One of the standout features is their ability to achieve tensile strengths ranging from 850 MPa to 1,700 MPa.
Precipitation hardening involves a sequence of steps including: solution treatment to dissolve precipitates, rapid cooling to create a supersaturated solid solution, and aging to form finely dispersed precipitates. This results in increased strength, improved wear resistance, and heightened corrosion resistance. Precipitation-hardened stainless steels find extensive applications across diverse industries, from oil & gas to aerospace.
This article will discuss what precipitation-hardened stainless steel is, how it works, the process behind it, its advantages and disadvantages, and more.
What Is Precipitation-Hardening of Stainless Steel?
Precipitation hardening (PH) of stainless steels creates a family of alloys that contain: iron, chromium, and nickel. The addition of one or more of the elements: aluminum, copper, molybdenum, titanium, and niobium and heat treatment creates a precipitation-hardened steel. Through heat treatment, these steels offer an ideal blend of characteristics found in both martensitic and austenitic grades. Similar to martensitic grades, they can achieve significant strength through heat treatment, while also possessing the corrosion resistance inherent to austenitic stainless steels. The PH stainless steels have tensile strengths ranging from 850 MPa to 1,700 MPa, accompanied by yield strengths spanning from 520 MPa to over 1,500 MPa. Elongation levels range from 1 to 25%, and the option of cold working prior to aging can be employed to gain even greater strengths. Precipitation-hardened stainless steel alloys are offered in two main states: annealed (Condition A) and tempered (Condition C). In the annealed state, these alloys are relatively soft and malleable, with Rockwell hardness ranging from B75 to C20. After forming, parts can undergo age-hardening to achieve higher Rockwell values, typically ranging from C35 to C49.
What Is the History of Precipitation Hardening Stainless Steel?
The history of precipitation hardening stainless steel dates back to the early 20th century. In 1929, a pivotal discovery was made by William J. Kroll of Luxembourg, who harnessed titanium to pioneer the concept of precipitation hardening in stainless steel. Notably, Kroll is renowned for developing the Kroll process, which revolutionized the refining of titanium and zirconium.
Fast-forward to the 1940s when the application of precipitation hardening in metals gained momentum. The first-generation martensitic precipitation-hardened stainless steel was designed during this time. Subsequently, in 1948, Armco's pioneering work led to the inception of 17-4 PH steel. 17-4 PH steel found its niche in aircraft landing frames, fasteners, and engine components. The evolution of 15-5 PH stainless steel was spurred by a strategic reduction in chromium content and an augmentation in nickel content within the 17-4 PH steel matrix. In recent years, propelled by contemporary advancements in materials science the landscape of PH stainless steel has flourished. Notably, Ferrium® S53®, a product of this era, has demonstrated extraordinary capabilities. It secured its place in aerospace applications and highlighted the enduring progression of precipitation hardening of stainless steel.
How Does Precipitation Hardening Stainless Steel Work?
Precipitation hardening stainless steel operates through a controlled heat treatment process. It starts with a solution treatment with which the alloy is heated to form a uniform solid solution. Rapid cooling, or quenching, follows, trapping alloying elements within the crystal structure. During aging, reheating causes these elements to precipitate as fine particles, hindering dislocation movement and increasing strength. Optional cold working before aging can enhance properties. The result is improved mechanical properties such as higher strength and hardness, achieved by the interaction between precipitates and dislocations.
What Is the Purpose of Precipitation Hardening Stainless Steel?
The primary purpose of PH stainless steel is to offer a material that combines the strengths of austenitic and martensitic stainless steels. It aims to provide exceptional strength, tailored properties, and corrosion resistance for demanding applications. Through a controlled heat treatment process, PH stainless steel achieves improved strength by forming fine precipitates within its microstructure. This process allows engineers to customize the material's properties to meet specific needs, striking a balance between strength, ductility, toughness, and corrosion resistance. It finds utility in diverse industries like: aerospace, marine, medical, and automotive, offering enhanced performance and durability.
What Are the Phases Involved in the Process of Precipitation Hardening Stainless Steel?
The key characteristic of precipitation-hardened stainless steels is their ability to undergo a series of metallurgical changes during the heat treatment process, which is illustrated in Figure 1:
Precipitation-hardening process.
Image Credit: https://www.ulbrich.com/blog/precipitation-hardening-stainless-steel-alloys-guide/
Here's a general overview of how the process works:
1. Solutionizing
Solutionizing, also known as "solution treatment," initiates the precipitation-hardening process. This step involves dissolving precipitates and minimizing potential alloy segregation. To achieve this, the material is heated to its solvus temperature and held there to foster the development of a uniform solid solution. Once this uniformity is achieved, the material is taken away from the heat source in readiness for the subsequent phase.
2. Quenching
The subsequent stage of the procedure involves swift cooling or quenching of the alloy. Within this phase, the material's cooling rate is so rapid that it results in a supersaturated solid solution containing surplus copper constituents. This swift transformation prohibits the diffusion of nucleation sites. It causes the quenching to occur so swiftly that precipitates are unable to form on the alloy.
3. Aging
The aging stage is the final stage in the precipitation-hardening process. Within this step, the material is subjected to further heating, but this time below the solvus temperature. This controlled heating prompts atoms to undergo limited diffusion over short distances. It results in the formation of finely dispersed precipitate layers within the material. This process effectively reinforces the alloy by restricting dislocation movement.
What Are the Requirements of Precipitation Hardening Stainless Steel?
Precipitation-hardened stainless steels are characterized by specific requirements that render them suitable for diverse applications. Firstly, their alloy composition includes elements like: iron, chromium, nickel, and additional additives such as: copper, aluminum, and titanium. These constituents are pivotal in enabling the process of precipitation hardening. This allows the material to achieve a desired equilibrium of strength and resistance to corrosion. Another fundamental requirement is adherence to a well-defined heat treatment procedure. It involves a sequence of steps like: solution treatment, quenching, and aging. This sequence of thermal treatments is essential to attain the desired microstructure and mechanical properties, with variations based on the specific alloy composition. Achieving an optimal balance between high strength and toughness is a critical consideration, ensuring that the material can withstand loads and impacts without suffering catastrophic failure. Equally crucial is the weldability of these steels, which must be tolerant of common welding methods to prevent issues such as: cracking, loss of mechanical properties, and compromised corrosion resistance in heat-affected zones.
How Long Does Precipitation Hardening Stainless Steel Take?
Precipitation hardening is a very sluggish process. The process of precipitating solid materials initiates by subjecting them to elevated temperatures for dissolution. This can last anywhere from an hour to a maximum of 20 hours. The resulting mixture can become highly saturated and readily amenable to further treatment such as quenching, subsequent aging, and artificial aging. A soaking period of two to 20 hours follows. The combination of soaking time and aging temperature is determined by the desired strength for the end product. Precise calculations of time and temperature are paramount. Excessive values can lead to reduced precipitates, resulting in higher ductility and lower strength. An alternate method for achieving precipitate hardening is natural aging, which, however, demands a longer time frame, ranging from days to weeks. The presence of impurities generated during the precipitation or hardening process can disrupt the crystal lattice structure in various materials, including: aluminum, stainless steel, and other alloys. Table 1 below shows the hardening temperatures and time for some alloys:
Type | Hardening Temperature and Time |
---|---|
Type H900 | Hardening Temperature and Time 482 °C, 1 hour |
Type H925 | Hardening Temperature and Time 496 °C, 4 hours |
Type H1025 | Hardening Temperature and Time 552 °C, 4 hours |
Type H1075 | Hardening Temperature and Time 580 °C, 4 hours |
Type H1100 | Hardening Temperature and Time 593 °C, 4 hours |
Type H1150 | Hardening Temperature and Time 621 °C, 4 hours |
Table Credit: https://www.azom.com/article.aspx?ArticleID=2819
At What Temperature Does Precipitation Hardening of Stainless Steel Occur?
It depends on the different stainless steel types and the desired outcomes. Martensitic PH steels, with 17-4 PH being a common example, undergo a transformation to martensite at relatively low temperatures, approximately 250 °C. Subsequent aging between 480 °C and 620 °C further enhances their strength.
On the other hand, austenitic-martensitic PH steels are initially fully austenitic after a solution treatment. To induce martensite, a secondary heat treatment at 750 °C for two hours followed by cooling to room temperature is necessary. For certain alloys, refrigeration at temperatures as low as -50 °C to -60 °C for eight hours may be needed to achieve a stable austenitic/martensitic structure.
What Are the Different Types of Precipitation-Hardened Stainless Steel?
The characterization of precipitation-hardened stainless steels is based on their final microstructures following heat treatment. They can be categorized into four distinct groups as listed and discussed below:
1. Semi-Austenitic PH Stainless Steels
Upon rapid cooling from the annealing temperature to room temperature, the semi-austenitic PH steels retain their austenitic structure. This property imparts favorable toughness and ductility for cold-forming processes, making them preferable over martensitic PH steels that tend to be excessively hard.
To induce hardening and strengthening, an initial transformation from austenite to martensite is necessary. This primes the material for subsequent treatment at the aging temperature. Heating semi-austenitic PH steels to the range of 650–870 °C prompts the precipitation of carbides. This process reduces the presence of austenite-stabilizing elements in the matrix, allowing partial transformation to martensite upon cooling to room temperature. Partial martensite transformation can also be achieved by refrigeration below the Ms temperature (the beginning of martensite transformation) or through cold working.
Consequently, the reinforcement of semi-austenitic PH stainless steels involves a dual-phase or two-step approach. Following the initial treatment that fosters martensite formation, the second phase involves exposure to the aging temperature of 455–593 °C. This exposure leads to precipitation, resulting in hardness and overall strengthening.
2. Austenitic PH Stainless Steels
Austenitic alloys maintain their austenitic structures through annealing and subsequent hardening via aging. At the annealing temperature of 1,095–1,120 °C, the precipitation hardening phase dissolves and remains in solution during rapid cooling. When these alloys are reheated within the range of 650 to 760 °C, precipitation occurs, resulting in heightened hardness and strength. Notably, the hardness of austenitic alloys remains below that of martensitic or semi-austenitic counterparts, and these alloys retain their non-magnetic properties.
3. Welding PH Stainless Steels
During welding operations, the heat introduced will inevitably induce areas of solution-treated or annealed base metal. To achieve the desired hardness in these zones, post-weld heat treatments may be necessary, involving either a single or double heat treatment approach. It is advisable to seek guidance from the steel producer or consult relevant literature for recommended welding practices and subsequent treatment protocols specific to the proprietary PH grade in question.
Thinner PH stainless steel sections generally do not require preheating before welding. The martensitic PH grades, distinguished by low-carbon content, do not undergo full hardening akin to martensitic stainless steels like Type 410.
In cases of single-pass welds, both the weld metal and the heat-affected zones tend to uniformly respond to post-weld precipitation hardening treatments. However, multiple pass welds display less uniformity. This leads to notable variations in the structure of the base metal, heat-affected zones, and weld metal. Annealing subsequent to welding promotes a more consistent structure, enabling a uniform response to ensuing precipitation hardening treatments.
For example, when welding a 17-4 PH plate under four inches in thickness, preheating isn't mandatory, but interpass temperatures up to 150 °C are commonly specified. For 17-4 PH plate thicknesses exceeding four inches, preheating to 95 °C and maintaining an interpass temperature of 95–260 °C is often deemed necessary for numerous applications. When welding 17-4 PH using bare ER630 wire, employ argon for GMAW welding or helium or argon gas for GTAW welding.
4. Martensitic PH Stainless Steels
Martensitic alloys, in particular, display primarily austenitic structures at annealing temperatures ranging from approximately 1,040 to 1,065 °C. Upon cooling to room temperature, these alloys undergo a transformative process that converts the austenite structure into martensite. Swift cooling in air or oil following this phase preserves the presence of additives like copper and columbium within the solid solution at ambient temperatures. In the temperature span of roughly 150 °C to room temperature, a transformation from austenite to martensite occurs. When the highly supersaturated solid solution in the martensite matrix is reheated to the aging temperature of 482 °C to 593 °C, minute particles precipitate, leading to heightened hardness and strength.
What Are the Uses of Precipitation-Hardened Stainless Steel?
Precipitation-hardened steel finds widespread industrial applications in various sectors.
In the oil & gas sector, precipitation hardening of steel is utilized for hardening gates, valves, and machinery components, as well as retaining rings, spring holders, and springs. In the automotive sector, it is used for strengthening engine parts, gears, shafts, balls, plungers, and bushings, along with chains, valves, and gears. PH steel is applied to aircraft components, airplane engine parts, and turbine blades. It is also employed in diverse applications including: valve stems, molding dies, processing equipment, fasteners, nuclear waste containers including pressure vessels, and seals in general industrial use.
Is Precipitation-Hardened Stainless Steel Used in the Aerospace Industry?
Yes, precipitation-hardened stainless steel is commonly used in the aerospace industry. Aerospace components subjected to high stress, extreme temperatures, and harsh environments benefit from the enhanced strength and corrosion resistance offered by precipitation-hardened stainless steel. Aircraft parts such as turbine blades, engine components, landing gear, fasteners, structural elements, and other critical components often incorporate precipitation-hardened stainless steel grades to ensure optimal performance and safety.
What Metals Can Be Precipitation Hardened Beside Stainless Steel?
A variety of metals beyond stainless steel can undergo precipitation hardening, enhancing their mechanical properties for specific applications. Some notable examples include:
1. Magnesium
Precipitation hardening is employed in certain magnesium alloys to improve their strength and performance, particularly in industries such as aerospace and automotive.
2. Titanium
Titanium alloys can also be subjected to precipitation-hardening processes to enhance their mechanical properties, making them suitable for aerospace, medical, and other demanding applications. To learn more, see our guide on What is Titanium.
3. Steels
Apart from stainless steel, other steel alloys can undergo precipitation hardening. This technique can be employed to tailor the mechanical properties of specific steel grades, such as high-strength low-alloy (HSLA) steels. To learn more, see our guide on the Components of Steel.
4. Aluminum Alloys
Similar to stainless steel, various aluminum alloys can be precipitation hardened to improve their strength and durability. This is especially common in aerospace and structural applications. To learn more, see our guide on Aluminum Alloy Properties.
5. Nickel
Certain nickel-based alloys, often used in high-temperature and corrosive environments, can undergo precipitation hardening to enhance their resistance to both mechanical and environmental stressors.
How Much Does a Precipitation-Hardened Stainless Steel Cost?
The cost of precipitation hardening stainless steel can vary widely depending on factors such as: the specific alloy composition, the quantity being purchased, market demand, supplier pricing, and regional factors. Generally, precipitation-hardened stainless steel tends to be more expensive than standard austenitic stainless steel due to its specialized properties and manufacturing processes.
The addition of alloying elements like: copper, molybdenum, aluminum, and titanium, as well as the specific heat treatment processes involved in precipitation hardening, contribute to the higher cost of these materials. Precipitation-hardened stainless steel typically falls within the range of $700–$1,000/ton. For accurate and up-to-date pricing, it's recommended to contact stainless steel suppliers, distributors, or manufacturers and request quotes based on your specific requirements and quantities.
Do Precipitation-Hardening Costs Depend on the Metal Used?
Yes, the cost of precipitation hardening can vary based on the specific alloy or metal used. Different metals have varying raw material costs, availability of alloying elements, and manufacturing processes, all of which contribute to differences in pricing.
What Are the Advantages of Precipitation Hardening Stainless Steel?
The benefits of precipitation hardening are outlined and discussed below:
- Can remarkably amplify a metal’s strength by a factor of up to four. The method fosters the development of a sturdier microstructure within the metal, leading to heightened overall strength.
- Ductility can be improved through precipitation hardening. This augmented ductility fortifies precipitation-hardened metals against cracking and breakage, enhancing their overall resilience.
- Contributes to increased corrosion resistance in metals.
- Enhances the material's ability to withstand wear and tear by creating a harder microstructure.
What Are the Disadvantages of Precipitation Hardening Stainless Steel?
Despite its advantages, precipitation hardening does come with certain disadvantages that need consideration. These include:
- Excessive heating during precipitation hardening can lead to brittleness or susceptibility to cracking and warping when the metal is rapidly cooled after the hardening process.
- The intricate nature of the process necessitates specialized equipment and expertise. Consequently, it is typically reserved for high-end applications in which the benefits of increased strength justify the higher investment.
- The process of precipitation hardening consumes time, often spanning days or weeks for the metal to attain its peak strength.
- Necessitates specialized equipment that might not be universally available.
- In certain instances, achieving desired outcomes through precipitation hardening may require multiple intricate steps. This complexity can escalate both the costs and technical demands of the process, rendering it unfeasible for certain applications.
Can Precipitation Hardening Be Reversed?
Yes, the effects of precipitation hardening can be reversed through a process known as "overaging" or "overage annealing." Precipitation hardening involves the controlled formation of fine precipitates within a material's microstructure to increase its strength and hardness. However, these precipitates can continue to grow and coarsen over time. This can lead to a reduction in mechanical properties such as strength and hardness, and an increase in ductility.
By subjecting the material to elevated temperatures for an extended period, the coarsening of the precipitates can be encouraged, effectively reversing the strengthening process. This process is often referred to as "overaging" because it involves aging the material beyond the point at which its mechanical properties are optimized.
It's important to note that the specifics of overaging, including temperature and duration, will depend on the material's composition and the desired outcome. Overaging can be a deliberate step in certain applications for which a balance between strength, toughness, and other properties is desired.
Also, in some cases, the production methods could initiate the early onset of the final age-hardening phase. This can be counteracted, however, by subjecting the material to re-solution treatment before proceeding with subsequent manufacturing steps.
Is Precipitation Hardening the Same as Hardening?
No. Precipitation hardening and hardening are related but are distinct processes in materials science. Hardening is a general term used to describe the process of making a material, typically a metal or an alloy, harder by altering its microstructure through various methods. This often involves heating the material to a specific temperature and then rapidly cooling it, which can result in changes to its crystal structure and mechanical properties. Hardening can also involve introducing certain alloying elements to the material. Hardening can be achieved through processes like quenching and tempering in steel, during which the material is heated and then cooled rapidly (quenched) to lock in a hardened state, followed by controlled reheating (tempering) to achieve a balance between hardness and toughness.
Precipitation hardening, on the other hand, is a specific type of hardening process that involves the formation of finely dispersed precipitates within the material's microstructure. These precipitates, often on the nanoscale, contribute to increased strength and hardness. The process typically includes steps such as solution treatment (heating to dissolve alloying elements), quenching (rapid cooling to trap these elements in solution), and aging (controlled reheating to allow the precipitates to form). This process is commonly used in certain stainless steels, aluminum alloys, and other materials to achieve tailored mechanical properties.
Is 18-8 (Type 304) Stainless Steel Hardened?
No. Type 304 (18-8) is categorized as an austenitic steel characterized by at least 18% chromium and 8% nickel content, along with a maximum carbon content of 0.08%. This non-magnetic steel doesn't gain hardness through heat treatment; instead, achieving elevated tensile strengths requires cold working.
Summary
This article presented precipitation-hardening stainless steel, explained it, and discussed how it works and its advantages. To learn more about precipitation-hardening stainless steel, contact a Xometry representative.
Xometry provides a wide range of manufacturing capabilities and other value-added services for all of your prototyping and production needs. Visit our website to learn more or to request a free, no-obligation quote.
Copyright and Trademark Notices
- Ferrium® S53® is a registered trademark of QuesTek Innovations LLC.
Disclaimer
The content appearing on this webpage is for informational purposes only. Xometry makes no representation or warranty of any kind, be it expressed or implied, as to the accuracy, completeness, or validity of the information. Any performance parameters, geometric tolerances, specific design features, quality and types of materials, or processes should not be inferred to represent what will be delivered by third-party suppliers or manufacturers through Xometry’s network. Buyers seeking quotes for parts are responsible for defining the specific requirements for those parts. Please refer to our terms and conditions for more information.
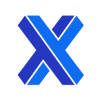