The term “metal” applies to a large portion of the periodic table of elements. Metals are typically characterized by high electrical and thermal conductivity, luster, and, in many cases, notable malleability, ductility, and tensile strength. Most are solid at room temperature and readily form metallic bonds. They are a core pillar of most product market segments thanks to their advantageous properties and broad versatility. Figure 1 below shows how metallic elements fit into the periodic table’s property trends.
This article will discuss metals, including their properties, uses, and types.
What Is Metal?
Metal elements are characterized by several distinct physical and chemical properties. They are typically found toward the left side of the periodic table and are distinguished by their ability to form positive ions (cations) via the loss of electrons. These cations form metallic bonds with free electrons, allowing them to move freely among the metal atoms. This bonding structure contributes to their unique properties, such as ductility, conductivity, and strength.
What Are the Different Properties of Metal?
The common properties of metals are listed and discussed below:
1. Density
Density is a fundamental physical property, quantifying mass per unit volume. Metals, on average, exhibit higher densities than non-metals, influenced by factors like atomic structure, atomic number/mass, and crystalline/atomic packing arrangement. Variations in composition, temperature, and pressure impact metal density; pure metals often boast higher densities than alloys.
2. Electronegativity
Electronegativity is a measure of an atom's electron-attracting capability when forming molecular bonds. In metals, it underpins their chemical behaviors and interactions. Metals typically exhibit low electronegativity compared to non-metals, signifying weaker electron attractions during bond formation. Consequently, metals readily donate electrons to nearby atoms, yielding positively charged ions (cations) that can then form ionic compounds or metallic bonds.
When forming bonds, metal ions are enveloped by a sea of delocalized electrons, enabling efficient electricity and heat conduction. Metals react with non-metals, forming ionic compounds with high melting points. Some such reactions generate impermeable oxide coatings like aluminum oxide or chromium oxide, while others, like iron oxide (rust), allow further degradation. Almost all metals oxidize readily when conditions allow.
3. Luster
Luster defines how light interacts with a material's surface and how well it reflects to the observer. Metals' electron configuration and metallic bonding enable strong electromagnetic (EM) reflection. Incident light interacts with delocalized electrons in the lattice. They’re easily absorbed and re-emitted, imparting a characteristic brilliance to the macroscopic surface.
Luster varies with surface finish, purity, and crystal structure, with polished surfaces exhibiting greater reflectivity. Beyond aesthetics, luster holds practical significance because it reflects infrared and radio frequencies in addition to visible light.
4. Malleability
Malleability denotes a material's ability to endure compressive stress and deform without fracturing. This trait defines metals’ utility and adaptability. Metallic bonding and regular crystalline structure enable metal atoms to glide over one another under pressure, remaining in lattice contact by delocalized electrons circulating among metal ions.
Malleability varies based on microscopic crystal structure, temperature, and purity. Metals with the closest-packed microstructure, like gold and silver, are the most malleable. Malleability facilitates forging, stamping, and rolling, among other processes.
5. Opacity
Metals’ electronic structure makes them opaque in the visible spectrum. When light interacts with a metal surface, it encounters free electrons, which absorb and scatter photons. This scattering prevents light from passing through in a straight line, resulting in opacity. Metals absorb many wavelengths of light, including the visible spectrum, so they’re highly opaque.
Overall, electronic structure, electron mobility, and light scattering within the lattice combine to make metals opaque at all visible frequencies and more or less reflective across most spectra. This property influences laser-based machining methods, thermal control/dissipation applications, and aesthetic roles.
6. Ductility
A metal’s intrinsic ductility determines how it can be formed into useful shapes. Metallic bonding fosters high ductility, as atomic layers slide over each other under stress. Ductility varies across metals, and it is affected by purity, crystalline structure, and other factors. More densely packed crystal structures, like those in gold and silver, show higher ductility.
This is a key property for metals drawn into wires, rods, or thin sheets for electrical wiring, cables, and metal foils. Ductility also enables intricate component shaping via forging, extrusion, and rolling.
7. Hardness
Hardness gauges a material's resistance to deformation, scratching, or penetration. In metals, it has functional significance, dictating the products’ durability. Standardized tests like Rockwell, Vickers, or Brinell measure hardness.
Atomic structure and bonding have the biggest influence on metal hardness — densely packed crystal structures like face-centered cubic (FCC) or body-centered cubic (BCC) translate into harder metals. Alloying elements, grain size, and heat treatment also impact hardness. Hardness guides material selection — hard metals find use in tools and wear-resistant coatings, while softer ones are critical in resilient structural components for buildings and vehicles.
8. Conductivity
Conductivity describes a material's ability to conduct electricity or heat. Both are crucial features in many metal applications.
The free electrons in a metal’s crystal structure enable excellent electrical current flow. This property is found in wiring, circuits, and electronics. For the same reason, metals generally exhibit high thermal conductivity, facilitating heat transfer via lattice vibrations and free electron movement. Applications span from heat exchangers to cooking utensils. Conductivity hinges on factors like crystal structure and purity; dense structures like copper and silver exhibit superior conductivity. These inherent conductive qualities are important factors in material selection for electronics and thermal management items.
9. High Tensile Strength
Tensile strength describes a material's ability to resist tensile forces without permanent deformation. It's key for structural and load-bearing applications, and metals are usually far stronger in tension than are polymers or ceramics.
Metallic bonding underpins this strength — the atoms shift elastically under tension. Tensile strength varies with factors like composition, crystal structure, grain size, and processing. Metals with greater crystallinity, smaller grains, and higher purity exhibit superior tensile strength.
10. High Reflectivity
Reflectivity defines a material's ability to reflect light or other electromagnetic radiation. It is essentially the same as luster but applies to a larger frequency range, from X-ray/UV to long-wave radio. Nevertheless, the mechanisms of reflectivity are the same as those describing luster.
11. Sonorousness
Sonorousness characterizes a material's ability to produce resonant sound upon impact, a hallmark of most metals. This property stems from the fact that sound waves propagate easily through metallic crystalline structures. Sound reflection further extends the sound effects. Striking a metal object, like a bell or plate, induces highly elastic vibrations that travel through the lattice, resulting in clear, ringing tones. The macro effect is shape oscillations that induce sound waves in the surrounding air.
Practical applications abound, particularly in musical instruments where metals produce rich, resonant sounds. Additionally, sonorous metals are used in acoustic engineering, enhancing sound reflection and architectural acoustics in buildings and performance spaces.
12. High Melting and Boiling Points
Most metals exhibit very high melting and boiling points (MP and BP). The most notable exceptions are lead, tin, gallium, and mercury. Metallic bonding is responsible for these characteristics, with variations in atomic size and packing density affecting specific values.
Tungsten and molybdenum have high MPs and may be added as alloying agents to raise the MP of various materials for high-temperature machinery. Tantalum also has a high BP, benefiting vacuum systems.
13. Corrosion Resistance
Corrosion resistance describes the material’s durability amid exposure to corrosive elements like moisture, chlorides, acids, and alkalis. It safeguards structural integrity and aesthetics for all types of products.
Metals can corrode via oxidation or other chemical reactions, leading to surface defects and weakened structures. Factors like composition, treatments, and environment influence resistance.
Stainless steel, nickel, chromium, zinc, aluminum, and titanium excel because they form impermeable and self-healing oxide layers on their surfaces. Many cheaper but high-performing metals, like steel, require coatings or alloying. Steel can be coated in zinc or alloyed with chromium to resist oxidation. Gold and silver are both highly resistant to corrosion under typical conditions, with gold being virtually immune to most corrosive agents.
Our Senior Solutions Engineer, Jacob Thompson, said the following, "One of our most commonly manufactured materials, in both CNC and Sheet Metal applications, is Stainless Steel 304. SS304 offers an excellent balance of corrosion resistance, strength, and affordability, making it suitable for a broad range of applications. Its austenitic structure allows for good formability and weldability, which simplifies fabrication. Additionally, its widespread availability and compliance with food-grade standards contribute to its popularity in industries like construction, food processing, and chemical equipment."
14. Magnetic Properties
Metals’ magnetic properties arise from their electron configuration and atomic arrangement. They are categorized into three types: ferromagnetic, paramagnetic, and diamagnetic metals. Ferromagnetic metals exhibit strong magnetic responses to external magnetic fields and will retain their magnetic alignment after being exposed to a magnetic field. Paramagnetic metals, on the other hand, are weakly attracted to magnetic fields but lose magnetization once the field is removed. Diamagnetic metals are repelled by magnetic fields and possess no net magnetization.
These three properties enable applications in magnetic storage, electromagnets, magnetic shielding, magnetic braking, and MRI machines.
15. Solid State at Room Temperature
Metals typically remain solid at room temperature due to the robust metallic bonds between atoms. The arrangement and strength of metallic bonds, influenced by factors like valence electrons and atomic size, dictate the melting point.
What Is the Use of Metal?
Metals have a plethora of applications owing to their physical, mechanical, and chemical properties. They are ideal for structural applications like construction and transportation, enduring heavy loads and harsh loading conditions. Their electrical and thermal conductivity makes metals vital in electrical wiring, electronics, and heating/cooling systems. Metals' malleability and ductility allow easy shaping and fabrication. Some offer excellent corrosion resistance, ideal for outdoor, marine, and chemically aggressive environments. High recyclability means metals can be reused, reducing the environmental impact of primary extraction and waste disposal. Some metals are visually appealing enough for jewelry and architectural finishes. Metals' high melting and boiling points ensure they retain mechanical strength, demanding high-temperature applications.
What Are the Different Types of Metal?
Metal families are differentiated based on their chemical and physical properties, as well as their position in the periodic table. Some types of metal are listed and discussed below:
1. Transition Metals
Transition metals occupy the d-block of the periodic table (groups 3 to 12). They’re distinguished by their partially filled d-orbitals, which lead to unique chemical and physical properties.
Transition metals display various oxidation states, forming colorful compounds. Known for high melting points, density, strength, and conductivity, they find applications in alloys, catalysts, and magnets. Transition metals also serve vital roles in biological systems, functioning as enzyme cofactors and participating in electron transfer reactions. Nearly all sectors find value in transition metals.
2. Heavy Metals
Heavy metals possess high atomic weights and high densities and include lead (Pb), mercury (Hg), cadmium (Cd), arsenic (As), chromium (Cr), uranium (U), and nickel (Ni), among others. Living cells tend to bioaccumulate these metals, meaning that heavy metals can be toxic to both humans and the broader ecosystem.
Despite their toxicity, heavy metals serve vital industrial functions in manufacturing, electronics, and pigment production. Stringent regulations aim to curb their environmental release and limit human exposure, moderating far-reaching impacts on ecosystems and public health.
3. Alkaline Earth Metals
Alkaline earth metals, found in group 2 of the periodic table, include magnesium (Mg), calcium (Ca), barium (Ba), beryllium (Be), strontium (Sr), and radium (Ra). These metals are less reactive than alkali metals but still readily react with water and oxygen, forming +2 oxidation state ions. They conduct electricity well. Compared to alkali metals, they possess higher melting and boiling points. They react with oxygen to form oxides and with water to form hydroxides, often exhibiting alkaline (basic) properties.
4. Precious Metals
Precious metals have long been valued for their relative rarity, natural occurrence in metallic form, aesthetics, and workability. They’ve figured prominently throughout human history in currency, jewelry, and decorative arts. Key among them are gold (Au), silver (Ag), and the platinum group metals (platinum, palladium, rhodium, ruthenium, iridium, and osmium).
Gold's appeal stems from its luster, malleability, and resistance to corrosion, making it an enduring symbol of wealth. Silver's brilliance and conductivity are widely used in currency, industry, and electronics. The platinum group metals, prized for their rarity and exceptional properties, serve critical roles in catalysis, electronics, and jewelry.
5. Ferrous Metals
Ferrous (iron-based) metals boast strength, durability, and magnetic attributes. This category encompasses pure iron, steel, and cast iron alloys.
Pure iron (Fe) is soft and ductile, and finds niche uses in wiring, magnets, and magnetic field guides. Steel is an iron-carbon alloy that regularly receives additions such as manganese, chromium, and nickel. Ferrous alloys are valued for strength, hardness, and other properties. Cast iron has a higher carbon content than steel and tends to be brittle, but is ideal for cast items like engine blocks and cookware, where the brittleness can be compensated by bulk.
6. Lanthanides
The lanthanides, also termed rare earth elements, make up the f-block of the periodic table from lanthanum (La) to lutetium (Lu). These elements share similar chemical properties and electron configurations, with lustrous silvery appearances and high melting and boiling points.
Reactive in nature, lanthanides easily form compounds with oxygen, water, and acids, which gives them extensive industrial utility. They're integral in catalyst production, magnets, lighting materials (phosphors), lasers, and batteries. Lanthanides also appear in glass, ceramics, medical imaging, medications, and cancer treatments.
Lanthanides' versatility makes them indispensable across electronics, energy, healthcare, and environmental sectors. Despite their abundance in the Earth's crust, their extraction and refinement can cause environmental problems like radioactive waste and chemical runoff.
7. Rare Earth Metals
The term “rare earth metals” is just another descriptor for the lanthanide group. Rare earths are sub-categorized into light (from lanthanum to samarium) and heavy (europium to lutetium).
8. Noble Metals
Noble metals are a group of metallic elements known for their resistance to corrosion, oxidation, and chemical reactivity under normal conditions. These metals are typically found in the transition metal group of the periodic table and include gold, silver, platinum, and palladium, among others. Key properties include chemical stability, high melting and boiling points, high electrical conductivity, catalytic properties, and biocompatibility.
9. Actinides
The actinide group, occupying the f-block beneath the lanthanide series in the periodic table, encompasses 15 elements from actinium (Ac) to lawrencium (Lr), notably including uranium and plutonium (Pu). Distinguished by their inherent radioactivity, all actinides emit radiation spontaneously as they decay into more stable forms. This necessitates careful management and disposal to mitigate health and environmental hazards. These concerns are particularly notable in mining contexts.
In terms of other properties, actinides’ chemical reactivity allows them to form various compounds, including oxides and complexes. Their isotopes have practical applications like nuclear fuel and smoke detectors. They are the sources of radioactive energy in nuclear reactors and weapons.
10. Base Metals
Older classification systems used the term “base metals” to distinguish some of the more common types from precious or noble metals. These abundant metallic elements are crucial for a wide spectrum of industrial applications. In the modern understanding of chemistry, base metals are not an official category. Instead, different industries apply them differently to use them as a general shorthand.
Some industrial contexts include iron in this group while others do not. More prominent base metals include copper, aluminum, zinc, nickel, lead, and tin.
Base metals are indispensable in construction, transportation, electronics, and manufacturing. They’re valued for their affordability, availability, and diversity of properties.
11. Non-Ferrous Metals
Non-ferrous metals, by definition, do not contain iron as the primary constituent. Unlike ferrous types, most non-ferrous metals exhibit superior corrosion resistance. Some combine low density and high strength and are ideal for the aerospace and automotive sectors. Other examples are highly conductive; copper and aluminum are essential in grid transmission wiring, electronics, and electrical systems. They are malleable and ductile and are highly recyclable. Examples are copper, aluminum, lead, zinc, nickel, chromium, tin, and the alloys thereof.
12. Light Metals
Light metals constitute a group known for their low density compared to ferrous or copper-based metals/alloys, making them invaluable in weight-sensitive applications. These metals are valued for their strength, durability, and lightness. Other properties include corrosion resistance, thermal and electrical conductivity, and recyclability.
Typical examples include aluminum, magnesium, titanium, and beryllium. They’re critical in the aerospace, automotive, electronics, and medical sectors.
13. Post-Transition Metals
Post-transition metals form a subset of elements positioned between transition metals and metalloids on the periodic table. Displaying properties that bridge the gap between transition metals and non-metals, they are notably softer and more ductile than transition metals and often generate covalent rather than metallic bonds. Their softness and ductility allow for easy shaping. Their lower melting and boiling points compared to transition metals facilitate easier manipulation. Because they react differently from most metals, they form an array of salts or other compounds with non-metals. Electrical and thermal conductivities are heavily influenced by individual properties and atomic structures, creating a wide range across the group.
Utilized in alloys, batteries, catalysts, and electronics, post-transition metals like aluminum, indium, tin, lead, and bismuth (Bi) play pivotal roles in various sectors.
14. Metalloids
Metalloids occupy a group of elements with properties that fall between those of metals and non-metals. Spanning from boron (B) to polonium (Po), they demonstrate traits like semiconductivity, conducting electricity better than non-metals but not as well as true metals. This makes them vital in semiconductors and electronics. They have varied physical traits but typically show a metallic luster and brittle character. Some behaviors lean more toward metallic traits, and others are more non-metallic, so metalloids show up in semiconductor manufacturing, glass production, and metallurgy.
Some are toxic, such as in the case of polonium, which is a strong 𝞪 (alpha) radiation emitter and has been used in high-profile assassinations.
Common examples include boron, silicon, germanium, arsenic, and antimony, serving pivotal roles in electronics, materials science, and everyday products.
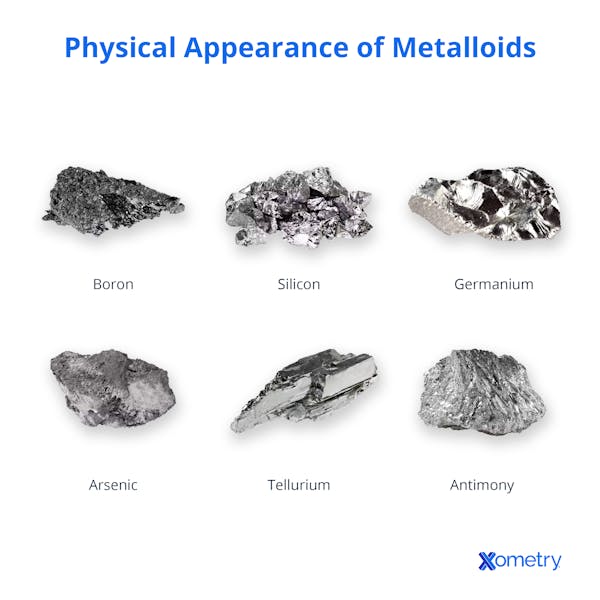
How To Choose Which Type of Metal To Use
To select the right metal, you must run through several critical steps:
- Identify the specific demands of the application, such as mechanical properties, environmental conditions, and budget constraints.
- Evaluate the desired properties (strength, conductivity, corrosion resistance, weight, etc.).
- List candidate metals that may meet the criteria.
- Consider the availability and supply chain robustness for suitable materials that meet your form, size, and quantity needs.
- Ensure compatibility with other materials and processes involved in the task.
- Consider the environmental impact of each metal, including factors such as recyclability, toxicity, and sustainability.
- Choose the most suitable metal based on your evaluation.
- Validate your selection through testing or analysis that is appropriate to the task.
What Type of Metal Can Be Used in 3D Printing?
Several metals are available for 3D printing processes, including:
- Stainless steel, which exhibits excellent mechanical properties, corrosion resistance, and versatility.
- Titanium is known for its strength-to-weight ratio, corrosion resistance, and biocompatibility, making it ideal for aerospace, medical, and dental applications.
- Aluminum and its alloys are lightweight yet strong metals used in the automotive, aerospace, and consumer electronics industries.
- Nickel-based alloys such as Inconel® and Hastelloy® are known for their heat resistance, corrosion resistance, and strength in extreme applications.
- Copper and copper alloys are used in electrical and thermal conductivity applications for electronics, heat exchangers, and plumbing systems.
- Tool steels are valued for their hardness, wear resistance, and toughness.
- Precious metals are used in jewelry, luxury goods, and electronics. Many are easy to form into intricate and customized shapes.
- Tungsten is known for its high melting point, density, and hardness in applications such as aerospace, defense, etc.
- Cobalt chromium alloys are strong, wear-resistant, and biocompatible, making them suitable for medical implants, dental restorations, and aerospace components.
To learn more, see our full guide on the 3D Printing Process.
What Type of Metal Can Be Used in Embossing?
Not all metals can be embossed. The following, however, are ductile enough for the process: copper and its alloys (bronze, brass, beryllium copper, etc.), steel, tinplate, nickel-plated steel, silver, and gold.
These metals offer a range of options for embossing, allowing for the creation of intricate designs and durable finishes. The best metal depends on the desired appearance, durability requirements, and budget considerations.
What Type of Metal Can Be Used in Laser Cutting?
Certain metals are well-suited to laser cutting, provided the laser is powerful enough, and it is the right type of laser. As affordable and available as it is, mild steel is one of the most common laser-cut metals. It cuts cleanly and quickly with minimal burrs. Stainless steel is another common one. It can be cut with a laser beam, producing precise edges and smooth surfaces. Aluminum is lightweight, corrosion-resistant, and easy to cut with a laser. It is very thermally conductive, which helps dissipate heat during cutting and reduces the risk of distortion. Other metals that can be laser cut include galvanized steel, copper, brass, and titanium.
To learn more, see our full guide on What is a Laser Cutter Used For.
What Type of Metal Can Be Used in Laser Engraving?
The laser engraving of metals is related to laser cutting, but it’s not exactly the same process. While many metals that can be laser cut can also be laser engraved, there are differences between the two processes that can impact the choice of metal.
Laser cutting typically requires metals that are thicker and requires a focus on clean cuts with minimal distortion.
Laser engraving focuses on creating marks or designs on the surface of the metal, often using lower power settings compared to cutting.
While most metals that can be laser cut can also be laser engraved, certain metals that are more reflective (like gold, silver, or aluminum) can present challenges in engraving due to their reflectivity, which can deflect the laser beam and cause inconsistent results.
Some metals that are difficult to cut (like harder steels or stainless steel) can still be engraved, but the engraving process may require more specialized settings or additional preparation, such as the application of a coating on the surface.
To learn more, see our full How Does a Laser Engraver Work guide.
What Is the Advantage of Using Metal?
Metals are inherently strong, durable, and versatile materials, making them suitable for a wide range of applications. When correctly applied, they provide structural integrity, resistance to wear, and the ability to withstand harsh environmental conditions. Metals also offer a diverse range of properties, including conductivity, fire resistance, and aesthetic appeal.
Additionally, metals are highly recyclable, contributing to sustainability efforts and reducing environmental impact. Overall, metal products tend to be cost-effective, enduring, and reliable.
What Is the Disadvantage of Using Metal?
Metals can be heavy, which may limit their use when weight is a concern. Additionally, some metals are prone to corrosion, requiring protective coatings or regular maintenance to prevent degradation. Certain metals — especially alloys with specialized properties — tend to be expensive. They can also be challenging to shape and form, requiring specialized equipment and processes.
Are Metals Generally Malleable and Ductile?
Yes, many metals are generally malleable and ductile which means they can be easily shaped or stretched without breaking. These properties of metals contribute to their ability to withstand mechanical stresses and provide structural integrity in engineering and construction applications. However, the degree of malleability and ductility can vary depending on the metal's type and composition.
Summary
This article presented metal, explained it, and discussed its properties and types. To learn more about metal, contact a Xometry representative.
Xometry provides a wide range of manufacturing capabilities and other value-added services for all of your prototyping and production needs. Visit our website to learn more or to request a free, no-obligation quote.
Copyright and Trademark Notices
- FDM® is a registered trademark of Special Metals Corporation
- Hastelloy® is a registered trademark of Haynes International Inc.
Disclaimer
The content appearing on this webpage is for informational purposes only. Xometry makes no representation or warranty of any kind, be it expressed or implied, as to the accuracy, completeness, or validity of the information. Any performance parameters, geometric tolerances, specific design features, quality and types of materials, or processes should not be inferred to represent what will be delivered by third-party suppliers or manufacturers through Xometry’s network. Buyers seeking quotes for parts are responsible for defining the specific requirements for those parts. Please refer to our terms and conditions for more information.
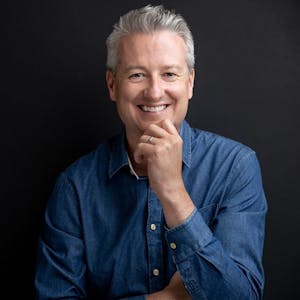