3D bioprinting represents a group of early-stage technologies. These fields of research examine the use of biological materials in printing functional implants and test devices that simulate, stimulate, or replicate real tissues, for either patient implants or research tools. While these technologies are at a very early stage, they show promise of paradigm shifts in medical interventions that have dramatic and far-reaching implications.
This article will discuss: What is 3D bioprinting?, its history, how it works, and its types.
What Is Meant by 3D Bioprinting?
3D bioprinting is the use of biological and bio-functional materials in additive manufacturing. Highly specialist printers are used to create 3D structures made of these biological materials. Some examples are: living cells, bio-active framework or scaffold materials, and biomolecules. The process uses typical 3D printing methods to deposit the biological material in layers, resulting in biological mimic, framework, and substitute constructions for diverse medical purposes.
The purpose of this 3D bioprinting is the manufacture of highly functional, complex tissue constructs and eventually organs. These are used for medical purposes such as patient implantation, drug testing, and pathology modeling. This technology is currently operating at fairly primitive levels. In terms of functioning tissues, however, research progress suggests it will revolutionize healthcare by enabling the custom manufacture of organs that are functionally similar to (or better than) the natural tissues they replicate.
When Did 3D Bioprinting Begin?
There is no single moment when the technologies and research that have resulted in 3D bioprinting suddenly broke through into patient solutions. However, several significant events stand out as seminal in defining the foundations of this technology. Gabor Forgacs observed that cells could be organized into “new” spatial structures and that they would combine and retain the structure indefinitely. This understanding was later key to the 3D construction of biological structures, as it taught that they could be induced to retain a shape.
Biocompatible materials began to be used in regenerative healthcare solutions around 2000. This led directly to the construction of spatial scaffolds, developed at Wake Forest University. Scaffolds were colonized with cultured patient cells, and implanted without rejection or immunosuppression drugs. These proved to be long-term stable. In 2002, bio-extrusion technology was reported by Landers, and commercialized as “3D-Bioplotter”. Wilson and Boland used a modified HP inkjet printer as a bioprinter in 2003 and then in 2004 developed cell-loaded bioprinting with a commercial SLA printer constructing scaffolds.
How Does 3D Bioprinting Work?
The process of 3D bioprinting generally consists of these steps:
- Create a 3D design of the tissues or organs that will be printed. Tools such as BioPrint Pro from Allevi 3D are developing fast.
- Select the ideal bio-ink. The material used in 3D printing contains materials such as: proteins and growth factors in biocompatible, photocured resins. These are off-the-shelf materials, ready to print inappropriate SLA bioprinter equipment. Before printing, they must be infused with cultured patient cells which will be stimulated to “grow” the organ.
- The bioprinter builds the model as designed, processing it through standard slicer software. Bio-inks are formulated for various production methods, such as extrusion, inkjet, and SLA printing. The deposited bio-ink fuses to form a porous structure, ready for cell maturation.
- The printed structure is cured to a more stable, cross-linked form by a variety of processes, suited to particular bio-ink types.
- After cross-linking, the printed material is incubated in a bioreactor. The printed tissue/organ will be treated as a living thing in this process, to optimize its development.
For more information, see our guide on How 3D Printers Work.
What Is the Importance of 3D Bioprinting?
The increasing use of bioprinting in all areas of patient care, drug development, and research is a result of the development of an increasingly powerful tool kit. This is the early stage of what is likely to become the manufacture of full replacement tissues and organs. The ability to custom-build new organs for surgical implants is on the verge of revolutionizing the entire medical sector. It allows patient treatments with the patient's tissues induced to deliver new, perfect, and “real” transplants with little or no rejection risk. Figure 1 is an example of a bioprinted organ:
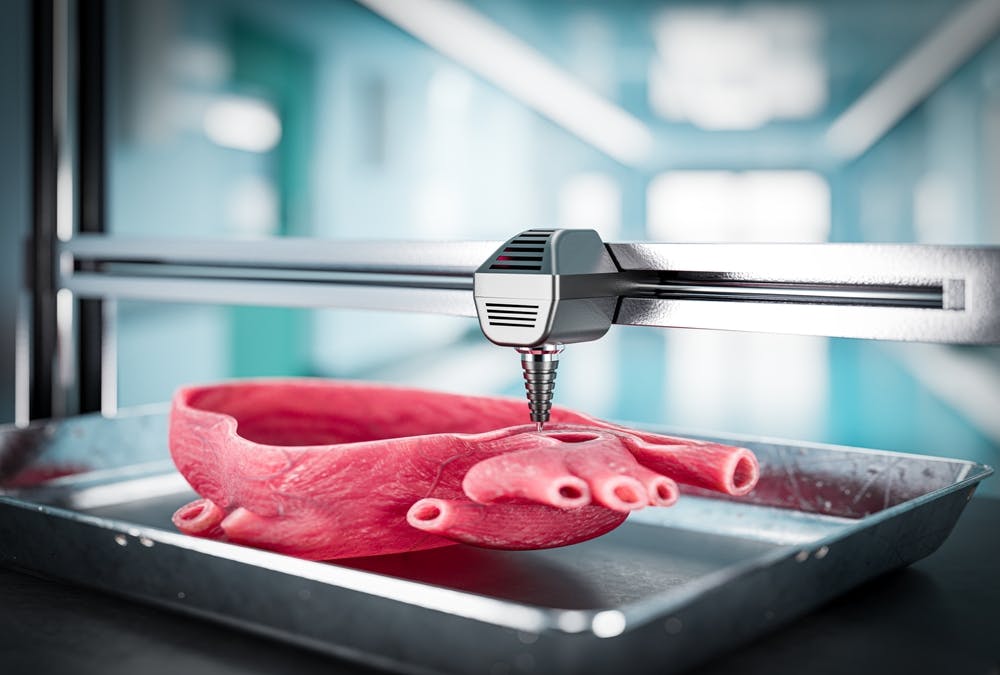
3D bioprinted organ.
Image Credit: Shutterstock.com/guteksk7
What Is the Purpose of 3D Bioprinting?
Bioprinting is the process of delivering incubation-ready, patient-cell-populated scaffolds that can be incubated and nurtured to become replacement organs. It is an irreplaceable, key step in delivering patient-ready transplantable tissues and organs that the patient's immune system will recognize as “self.”
What Are the Different Types of 3D Bioprinting?
Bioprinting has developed in three distinct strands, each with its difficulties and benefits:
1. Inkjet-based Bioprinting
Inkjet-based bioprinting uses specifically modified inkjet printing to place living cells and biomaterials onto a stereolithographic 3D construct to build biological structures—tissues and organs. The printhead dispenses bio-ink containing patient cells and biological support media, delivering a “picture” of each slice in the 3D design, built on the prior layer. These bio-inks contain UV-cured or heat-hardened elements that integrate and bond each layer to the one below. By this means, the tissues as designed are laid out into a 3D structure that can then be incubated to maturity.
Inkjet-based bioprinting is high resolution, high speed, and suited to applying multiple cell types or biomaterials in one print. While far from mainstream as yet, this technology is a key experimental method in tissue engineering for regenerative and implant medicine and also in drug testing.
2. Pressure-Assisted Bioprinting
Pressure-assisted bioprinting uses a pneumatic- or hydraulic-driven delivery of fine droplets of bio-ink onto a build platform. This constructs the tissues as designed, in a layer-by-layer process. When the bio-ink layer is deposited, it is cured through ultraviolet light exposure or temperature change. This process helps make an integral structure that can be incubated to mature the patient (or research test) ready tissues. This process is simpler in many regards than the alternatives. It allows mixed cell placement, for closer mimicry of real tissues. The resolution is lower, being extruded-droplet-based. In many cases, this is a small disadvantage to an otherwise powerful tissue construction method.
3. Laser-Assisted Bioprinting
Laser-assisted bioprinting uses a laser to transfer and precisely deposit living cells or biomaterials onto a build platform. It creates the desired 3D biological structures, such as tissues and organs. The laser beam evaporates substrate material on a bio-ink-loaded transfer tape. This causes the substrate to flash evaporate and eject the bio-ink onto the build. This deposits precise droplets of bio-ink onto the build layer-by-layer to create the desired 3D structure. This is a close simulation of bubble jet printing.
Laser-based bioprinting offers various benefits over other 3D printing techniques, including high-precision control over cell placement, the ability to print with high resolution, and the ability to use a range of biomaterials, including those with more complex compositions. Excess laser power can result in cell damage, and the technique is poorly equipped to deliver high cell densities.
What Are the Different 3D Bioprinting Approaches?
The different 3D bioprinting approaches are listed below:
1. Biomimicry
Biomimicry uses natural tissue processes and materials to solve structural and functional bioprinting problems. Biomimicry can create more effective methods for producing closely analogous biological tissues and organs. The use of natural extracellular matrix (ECM) materials to create scaffolds for tissue engineering is one form of biomimicry. ECM provides structural support to cells. Using natural ECM materials like collagen and hyaluronic acid can improve the structural integrity and functionality of printed tissue outcomes.
Using bio-inks that contain non-natural materials that mimic the properties of natural tissues, such as stiffness, elasticity, and cell adhesion, can help to create better-functioning printed tissues. Some researchers are exploring 3D printing methods that mimic the way spiders spin webs to create complex and more nature-typical properties in printed biological structures.
2. Autonomous Self-Assembly
Autonomous self-assembly seeks to enable cells to self-organize and form the required structures without the need for direct manipulation/placement. This approach seeks to mimic the way cells naturally assemble, in the normal growth of tissues. Patient cells are mixed with a bio-ink that contains a gel material that can be molded into the required shape. The cells and bio-ink are then incubated to allow self-organization to happen. This forms tissue or organ structures that are closer to nature. This approach is different from traditional bioprinting methods, in which cells are precisely placed in a predetermined pattern to create a structure.
Autonomous self-assembly bioprinting has major advantages over traditional bioprinting if it can be achieved repeatably and made predictably. For example, it allows for the creation of more complex and realistic tissue structures by mobilizing developmental-morphogenetic processes. Removing the need for external manipulation reduces the cell damage that can be a barrier in bioprinting.
Researchers are developing new materials and techniques to guide the natural and intrinsic self-assembly process, to deliver a more natural and higher-functioning outcome. This is perhaps the most significant area of research in bioprinting, as it has the potential to bring about another revolution in tissue engineering and regenerative medicine.
3. Mini-Tissue
Mini-tissues (or microtissues) are limited three-dimensional cellular structures. These are used in drug discovery, toxicity testing, and tissue engineering—and specifically not as patient implants. They are printed by the generally used methods: normal bio-inks laced with living cells. The creation of mini-tissues offers advantages over traditional 2D, Petri dish cell cultures that are otherwise used. By more closely mimicking the complexity of natural tissues, they offer more realistic results for drug testing and toxicity screening. Researchers expect to assemble larger tissues and organs that can potentially be used as implants in patients by printing small building blocks.
What Are the Steps of the 3D Bioprinting Process?
Bioprinting is a process that requires rigor and high control in all regards. Each step below is an area of intense research, as alternative techniques and materials are constantly being developed:
1. Pre-Bioprinting
The pre-bioprinting process consists of various steps that must all be exactly right—margins for variance and error are minimal if the result is to be a useful tissue structure. The first step is to conceptualize and specify the desired structure. This will define the shape, size, and general physical properties of the tissue and the types of cells and support/nutrient materials that will be used. With the outline of the structure defined, specialist CAD tools are used to create a highly detailed 3D model of the structure. An appropriate bio-ink is then selected or created, containing the structural, hardening, and nutrient mix appropriate for the tissues to be grown. Cell selection and cultivation in vitro is the most delicate step, involving incubating and encouraging the reproduction of cells in a culture medium under tightly controlled conditions, to ensure their viability and sufficiency.
2. Bioprinting
Bioprinting is the final realization step that takes the foundational work and builds the required tissue sample. It is ready for incubation and the planned uses for drug evaluation, toxicity testing, or patient implant. The tissue sample is constructed either by stereolithographic methods or by the self-organization that was designed into the plan.
3. Post-Bioprinting
After bioprinting, there are various critical processing steps that ensure the function and viability of the built tissue. First, the printed matrix material must be cross-linked to create a robust and stable structure. Various methods are available, typically UV cure, heat treatment, and externally applied chemical agents. Maturation/incubation then allows the cells to divide and differentiate. Tightly controlled environmental conditions are required for this to proceed. During maturation, and again once matured, the viability of the cells is assessed to ensure they are performing their intended functions. After maturation, the built tissue is characterized to determine its physical, biological, and biochemical character. This process uses techniques such as histology or immunohistochemistry to assess the tissue behavior. Finally, the bioprinted tissue will be tested to ensure it is functioning as expected. A wide range of possible tests are available, suited to particular tissue types.
What Are the Applications of 3D Bioprinting?
Bioprinting has a growing list of applications, but the main functions it serves at this point are listed below:
- Drug performance and adverse reaction assessment.
- Toxicology testing.
- Patient implant.
What Are the Benefits of 3D Bioprinting?
Bioprinting is a powerful set of techniques that is enabling increasingly powerful capabilities across most areas of patient healthcare, drug development, environment, and toxicity testing. Listed below are some of its benefits:
- Allows the precision building of complex tissue structures.
- Can be used to create 3D models of organs for drug testing. This allows for faster and less ethics-restricted testing of drug formulations.
- Reduces the need for animal testing.
- Can create custom implants, tailored to fit a specific patient's needs.
- Can build living tissues and organs for transplant, though this capacity is limited to simple structures as yet. These will be built from the patient’s cells, so rejection is shown to be minimal.
What Are the Limitations of 3D Bioprinting?
Bioprinting has severe limitations that are the subject of extensive research, including:
- Cannot currently print complex tissues and organs with various cell types, blood vessels, and nerves.
- Bioprinting materials are expensive and difficult to produce.
- The mechanics of the printing processes often damage or destroy cells. This limits the viability of the printed tissues.
- Still an expensive and intensively lab-based technology. It uses expensive equipment and requires extraordinary skills.
- There are, as yet, no standards or widely accepted guidelines. Assessment of results between methods and research groups is challenging.
How Does 3D Bioprinting Drive Innovation in the Medical Industry?
Bioprinting is set to become the primary regenerative surgery tool for a wide spectrum of degenerative diseases and physiological conditions. Printing a new, functional heart to replace a patient's damaged one is still a distant prospect, but the early building blocks are in place. Right now, bioprinting is shortening and reducing the cost of drug evaluation and certification cycles. It reduces the barriers to market entry for novel pharmaceuticals.
What Type of 3D Printing is Bioprinting?
Bioprinting is generally based on stereolithographic methods, building tissues layer by layer from a 3D file. The next revolution is likely to move this more toward self-organization, at least in cellular distribution and positioning.
Are 3D Printing and 3D Bioprinting the Same?
No, 3D printing and 3D bioprinting are not the same, but there are areas of similarity. Some of the tools used in bioprinting are interchangeable with those in the rapid prototyping sector. However, the divergence between the two sectors is clear and is set to increase rapidly. Bioprinting is rapidly exceeding the complexity limitations of the rapid prototyping sector, as researchers seek to produce ever more complex and higher-functioning outcomes.
Summary
This article presented 3D bioprinting, explained it, and discussed its various types and applications. To learn more about 3D printing in other industries, contact a Xometry representative.
Xometry provides a wide range of manufacturing capabilities, including 3D printing and other value-added services for all of your prototyping and production needs. Visit our website to learn more or to request a free, no-obligation quote.
Disclaimer
The content appearing on this webpage is for informational purposes only. Xometry makes no representation or warranty of any kind, be it expressed or implied, as to the accuracy, completeness, or validity of the information. Any performance parameters, geometric tolerances, specific design features, quality and types of materials, or processes should not be inferred to represent what will be delivered by third-party suppliers or manufacturers through Xometry’s network. Buyers seeking quotes for parts are responsible for defining the specific requirements for those parts. Please refer to our terms and conditions for more information.
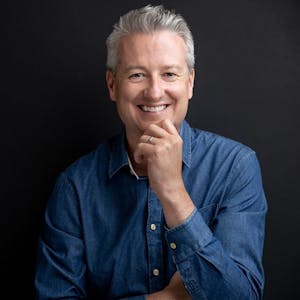