Harper-Dorn creep is a complex phenomenon that occurs in metals, alloys, ceramics, and even ice. Unlike other types of creep, Harper-Dorn creep operates at high temperatures and low stresses, making it a critical consideration in applications where these conditions are prevalent. This unique mechanism involves edge dislocations which can lead to deformation and strain accumulation over time.
To predict and mitigate Harper-Dorn creep, you must first understand the factors that contribute to it. Factors such as grain size, dislocation density, and material composition play key roles in determining the extent and rate of Harper-Dorn creep. In this article we will take a closer look at the definition of Harper-Dorn creep, the factors that influence it, and ways to reduce it.
What Is Harper–Dorn Creep?
Harper-Dorn creep is a high-temperature deformation mechanism that occurs in low-stress situations in certain metals, alloys, ceramics, and ice. It is characterized by a stress-dependent creep rate and a stress exponent that decreases from a high value to a value close to 1 as the stress diminishes. This phenomenon was first observed in aluminum and later found in other materials as well.
During Harper-Dorn creep, the dislocations move through the material, creating strain and contributing to creep deformation. The stress exponent represents the sensitivity of the creep rate to applied stress, with higher exponents indicating a stronger stress dependence.
The underlying mechanisms and factors influencing Harper-Dorn creep are still topics of research and debate. Some studies suggest that impurities, such as low levels of alloying elements, may play a role in facilitating Harper-Dorn creep. Other proposed mechanisms include dynamic recrystallization and grain growth. Full understanding of the precise mechanisms behind Harper-Dorn creep will only come with further research. For more information, see our guide on the Creep of Materials.
How Does Harper–Dorn Creep Occur?
Harper-Dorn creep occurs through a combination of dislocation glide and climb in the crystal lattice of metals and alloys at high temperatures. The general mechanisms involved are as follows:
- Dislocation Glide: Dislocations are linear defects in the crystal lattice that allow atoms to move with respect to each other. In Harper-Dorn creep, dislocations glide through the lattice planes, causing plastic deformation and strain in the material. This glide motion is driven by the applied stress.
- Dislocation Climb: Dislocation climb refers to the motion of dislocations perpendicular to the glide planes. It allows dislocations to overcome obstacles and move through the lattice in the direction of the applied stress. Climb is often facilitated by the diffusion of atoms, which helps dislocations move along their glide directions.
- Athermal Storage and Dynamic Recovery: The dislocation network undergoes continuous evolution during Harper-Dorn creep. Athermal storage refers to the accumulation of dislocations in the network due to applied stress. Dynamic recovery involves the annihilation of mobile dislocations with network dislocations, leading to local restoration of the dislocation structure.
- Static Recovery and Restoration: Static recovery refers to the restoration processes induced by stored line energy in the dislocation network. It can involve processes like dislocation annihilation and rearrangement, leading to a reduction in dislocation density and the creation of subgrains or recrystallized grains.
What Are the Factors That Contribute to Harper-Dorn Creep?
Several factors contribute to Harper-Dorn creep in metals and alloys. These factors can influence the likelihood, rate, and characteristics of the creep process:
- Temperature: Harper-Dorn creep typically occurs at high temperatures, often approaching or exceeding half of the material’s absolute melting temperature. Elevated temperatures facilitate atomic diffusion, making dislocation glide and climb more common and pronounced.
- Stress Level: Harper-Dorn creep is observed at low stresses, typically below a certain threshold. The exact stress level depends on the material and temperature. The stress level affects the rate of dislocation motion and the balance between glide and climb mechanisms.
- Material Purity: High-purity materials are more susceptible to Harper-Dorn creep than those with more impurities. The presence of impurities can affect dislocation mobility and alter the restoration mechanisms, potentially inhibiting or modifying creep behavior.
- Dislocation Density: Harper-Dorn creep is commonly associated with low dislocation densities. When the dislocation density is low, the dislocation motion and interaction are influenced more by the thermal activation of the dislocation climb, leading to the characteristic creep behavior.
- Microstructure: The microstructural characteristics of the material such as grain size, grain boundaries, and subgrains can influence Harper-Dorn creep. Fine-grained materials or materials with a well-defined array of subgrains may exhibit different creep behavior than coarse-grained materials.
- Restoration Mechanisms: The relative contributions of different restoration mechanisms such as athermal storage, dynamic recovery, and static recovery can impact the creep behavior. The dominant restoration mechanism may vary depending on the material, temperature, and stress conditions.
What Is the Importance of Harper–Dorn Creep in the Manufacturing Process?
Harper-Dorn creep has both practical and scientific importance in the manufacturing process. Understanding the creep behavior of materials is crucial for designing components that will be subjected to long-term stresses at elevated temperatures. By considering Harper-Dorn creep in component design, engineers can design materials and structures to withstand the expected service conditions without failing or excessively deforming.
For example, in the aerospace, power generation, and chemical processing industries, materials are often exposed to high temperatures for extended periods. Harper-Dorn creep can cause gradual deformation and dimensional changes in components, which can then compromise their functionality and structural integrity. By studying and accounting for Harper-Dorn creep, manufacturers can select appropriate materials and design strategies to mitigate its effects. Additionally, researchers can advance their knowledge of material properties and develop better models and theories to accurately predict creep behavior.
Who Authored Harper-Dorn Creep?
The Harper-Dorn creep mechanism was first discovered by Harper and Dorn in 1957, who worked at the University of California, Berkeley. While Harper and Dorn made notable individual contributions, the term Harper-Dorn creep refers to the collective understanding and combined influence of their research and others on creep behavior. Their research focused on high-temperature creep behavior, specifically the migration of the stress exponent (n-value) toward unity (n = 1) at very low applied stresses. The work of Harper and Dorn has significantly contributed to the understanding of creep deformation in metals and alloys.
How Can Harper–Dorn Creep Be Reduced?
Harper-Dorn creep in metals and alloys can be minimized through various approaches. Here are some strategies that can help mitigate Harper-Dorn creep:
- Alloying: The addition of alloying elements can alter the material's microstructure and improve its creep resistance. For example, elements like chromium, molybdenum, and tungsten can form stable carbides or intermetallic phases which hinder dislocation motion and enhance creep resistance.
- Grain Refinement: By refining the grain size of the material, you can impede dislocation movement and enhance creep resistance. Severe plastic deformation, grain boundary pinning, and rapid solidification methods can generate finer-grained microstructures, reducing Harper-Dorn creep.
- Heat Treatment: Appropriate heat treatment processes, such as solution treatment and aging, can optimize the material's microstructure and enhance its creep resistance. Precipitation hardening or the formation of dispersed second-phase particles can hinder dislocation motion and impede creep deformation.
- Texture Control: The crystallographic texture of the material influences its creep behavior. Techniques like directional solidification or thermomechanical processing will let you set a favorable, creep-resistant texture orientation.
- Stress Reduction: Try to minimize the applied stress level so that creep is not induced. Apply stress-relieving techniques, design components with lower stress concentrations, or operate the system at lower stress levels whenever feasible.
- Elevated Temperature Testing: Learn about your material’s creep behavior through rigorous testing at elevated temperatures and low stresses. This can help you identify the critical stress levels and temperatures at which Harper-Dorn creep becomes significant, enabling better design and material selection.
How Does Harper–Dorn Creep Compare to Dislocation Creep?
Both Harper-Dorn creep and dislocation creep occur in metals and alloys. While they both involve the movement of dislocations, the mechanisms, temperature and stress dependencies, strain rate sensitivities, and deformation modes of Harper-Dorn creep and dislocation creep differ significantly. Dislocation creep occurs at high temperatures and under high-stress conditions, driven by the motion and interaction of dislocations within the crystal lattice. Harper-Dorn creep, on the other hand, occurs at lower stresses and intermediate temperatures, primarily via diffusion along grain boundaries.
How Is the Harper–Dorn Creep Rate Derived?
The relationship between the applied stress and the steady-state creep rate in Harper-Dorn creep can be described by the following equation:
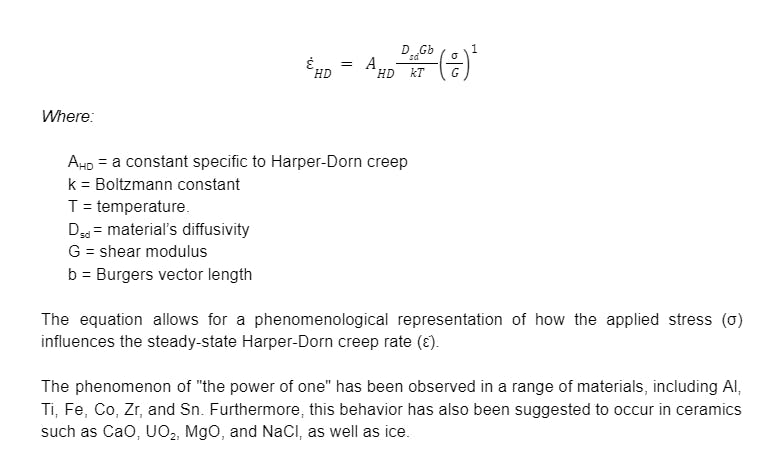
Harper-Dorn creep equation.
How Does Strain Impact on Harper-Dorn Creep?
The impact of strain on Harper-Dorn creep can vary depending on the specific material and conditions. In general, strain has a major impact on the material’s behavior. Here are some ways in which strain can impact Harper-Dorn creep:
- Strain Rate Sensitivity: Harper-Dorn creep exhibits strain rate sensitivity, meaning that the creep rate is influenced by the applied strain rate. Higher strain rates typically result in higher creep rates, indicating greater creep deformation under increased strain.
- Creep Strain Accumulation: Over time, Harper-Dorn creep leads to the accumulation of creep strain in the material. The strain gradually increases as creep deformation progresses, which can ultimately affect the material's mechanical properties and structural integrity.
- Stress-Strain Relationship: The relationship between stress and strain in Harper-Dorn creep can follow a power-law behavior. This equation connects the applied stress and the steady-state creep rate, integrating material characteristics and temperature as key factors. The strain is an integral part of this equation, influencing the overall creep behavior.
- Creep Rupture: Excessive strain under high-stress conditions can lead to creep rupture, where the material fails due to continuous deformation. Creep rupture is a critical failure mode in Harper-Dorn creep and is influenced by the applied strain and stress levels.
Does Harper–Dorn Creep Occur in Aluminum?
Yes, Harper-Dorn creep can occur in aluminum and its alloys under certain conditions. Both dislocation creep and diffusion creep mechanisms can be involved in this metal’s creep behavior. Harper-Dorn creep, which is a diffusion-controlled process, can manifest in aluminum when diffusion along grain boundaries becomes a dominant deformation mechanism.
The microstructure of aluminum, including the grain size, grain boundary characteristics, and presence of second-phase particles, can affect the extent of Harper-Dorn creep. Other factors such as temperature, stress levels, and alloy composition also contribute to aluminum’s susceptibility to Harper-Dorn creep. For more information, see our guide on What is Aluminum?
Is Harper–Dorn Creep Caused by High Temperature?
Yes, Harper-Dorn creep is typically associated with high temperatures. The phenomenon occurs when the temperature of a material is high enough to facilitate diffusion-controlled deformation. At elevated temperatures, atomic diffusion becomes more pronounced, allowing atoms to migrate and rearrange within the material.
However, it is important to note that while high temperature is a general requirement for Harper-Dorn creep, it is not the sole factor influencing its occurrence. Other parameters such as stress, grain size, and material composition also play significant roles in determining the susceptibility of a material to Harper-Dorn creep.
Is Harper–Dorn Creep Dependent on Grain Size?
Yes, grain size plays a crucial role in Harper-Dorn creep in metals and alloys. Grain boundaries act as barriers to the movement of dislocations. Dislocations encounter resistance as they navigate through the grain boundaries, impeding their glide and climb processes. A material with smaller grains has more such boundaries than one with large grains, so it will have more inherent resistance to dislocation creep. Additionally, larger grain sizes in materials promote grain boundary sliding as a deformation mechanism. With more extensive grain boundaries present, adjacent grains can slide along these boundaries, accommodating strain and contributing to creep deformation. Grain boundary sliding may have different implications for the overall creep behavior compared to dislocation-mediated mechanisms.
On the other hand, grain boundaries can serve as preferential paths for atomic diffusion. Finer grain sizes mean more grain boundaries, thereby enhancing diffusional processes across these boundaries. This can lead to the prominence of diffusion-assisted creep in fine-grained materials, thereby influencing the overall creep behavior. Additionally, in certain materials like aluminum, well-defined subgrains can form during the creep process. Subgrains are smaller regions within grains that possess distinct crystallographic orientations. The presence of subgrains can impact the motion and interactions of dislocations, potentially influencing the overall creep behavior.
What Is the Difference Between Harper–Dorn Creep and Nabarro-Herring Creep?
Harper-Dorn creep and Nabarro-Herring creep are two different mechanisms of creep deformation that occur in materials at high temperatures. Harper-Dorn creep has been observed in various metals, alloys, ceramics, and ice. It has been studied extensively in the past 20 years to understand its detailed mechanism. That research has confirmed that Harper-Dorn and Nabarro-Herring creep are independent processes, and the predominance of one over the other depends on the grain size. Harper-Dorn creep dominates if the grains are larger than a critical value, while Nabarro-Herring creep is dominant for smaller grain sizes.
The activation energy for Harper-Dorn creep is similar to that of self-diffusion, indicating that vacancy diffusion controls the creep rate in this mechanism. Dislocations are considered to be sources and sinks of vacancies. The dislocation density in Harper-Dorn creep remains relatively constant and is independent of the applied stress. The transition between Nabarro-Herring and Harper-Dorn creep occurs when the grain size exceeds a critical value and the dislocation density is low.
Summary
This article presented Harper-Dorn creep, explained it, and discussed its various factors and how to reduce it. To learn more about creep, contact a Xometry representative.
Xometry provides a wide range of manufacturing capabilities and other value-added services for all of your prototyping and production needs. Visit our website to learn more or to request a free, no-obligation quote.
Disclaimer
The content appearing on this webpage is for informational purposes only. Xometry makes no representation or warranty of any kind, be it expressed or implied, as to the accuracy, completeness, or validity of the information. Any performance parameters, geometric tolerances, specific design features, quality and types of materials, or processes should not be inferred to represent what will be delivered by third-party suppliers or manufacturers through Xometry’s network. Buyers seeking quotes for parts are responsible for defining the specific requirements for those parts. Please refer to our terms and conditions for more information.
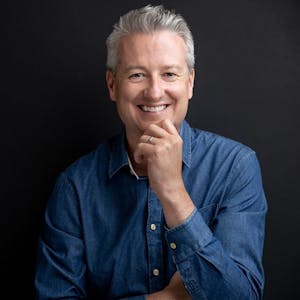