Tempering is a critically important process in the manufacture of heat-treated steel parts, particularly if high hardness is desired. The technique is an ancient one. It was developed by swordsmiths through the early Iron Age, as they forged and hardened Iron weapons in barely understood processes that made primitive steels. This article will discuss tempering, its definition, purpose, how it works, and the various stages.
What Is Tempering?
Tempering is the heat treatment process used in metallurgy (and also in glass) to improve the mechanical properties of the treated material. Mostly applied to steels, it involves reheating a previously hardened or quenched material to a specific temperature and then cooling it in a controlled manner.
Tempering is a critical step in optimizing the properties of products such as: tools, machine parts, and structural components, to achieve the desired balance between hardness and toughness. The temperature, heat duration, and cooling duration of tempering are closely controlled to achieve optimal properties, with specific parameters dependent on the material and its intended application.
What Is Tempering Also Known As?
Tempering is also referred to as "draw tempering" or simply "drawing". These terms are less commonly used. However, they still describe the heat treatment process of reheating quenched materials, typically steel, to a controlled temperature, followed by gradual cooling, to improve their mechanical properties.
What Is the History of Tempering?
The history of tempering is intertwined with the broader history of metallurgy and, in particular, the extremely complex and intricate history of the development of steel. Initial experience of tempering can be traced back to ancient civilizations. Metalworkers discovered, through both accident and experimentation, that heating and cooling metals could enhance their properties. During the European Iron Age and into the Middle Ages, blacksmiths gained a better understanding of tempering through trial and error. Highlights in this were the experiences of Toledo swordsmiths, who used wooden anvils to create exceptionally valued swords. They learned, by trial and error, that tempering improved their products by reducing brittleness and improving fracture properties. Japanese swordsmiths underwent a similar realization. Learning to control the tempering process achieved specific hardness and hugely improved toughness levels.
The 18th and 19th centuries brought gigantic advancements in metallurgy, alloy developments, and the understanding of heat treatment processes. Large-scale steel production and the development of standardized tempering procedures enabled better solutions to old and new problems. The move from wrought iron to tempered steel for boilers, for example, allowed higher steam pressures to be safely achieved. In the late 19th and early 20th centuries, microscopy and the onset of understanding the microstructure of steel changed the technology markedly. Precise comprehension of the effects of tempering temperature and time contributed to a deep understanding of the process as part of the heat treatment continuum.
What Is the Purpose of Tempering?
Tempering has become a fundamental process in metallurgy. It allows engineers and manufacturers to tune the mechanical properties of steel and other materials to meet the demands of an increasingly discerning and complex market.
Tempering reduces the extreme hardness achieved through quenching, making the material less brittle and more ductile. It increases material toughness, reducing fracture risk and making products more capable of withstanding the impact and stress of use (and abuse). Tempering tends to relieve internal stresses created during quenching. It reduces the risk of material distortion during machining or use and limits fracture initiation and propagation.
Why Is Tempering Important?
Tempering brings crucial benefits, without which whole classes of products would either be significantly less effective or in many cases, simply impossible. Tempering can remove much of the brittleness of quenched materials, making them less prone to fractures and better able to absorb impact and stress. The technique increases material ductility, allowing for greater deformation without breaking. It improves the survivability and functional applications of heat-treated steel (and other metal) products. Reducing or removing the internal stresses of a part after heat treatment reduces the risk of distortion occurring as a result of subsequent material removal processes. Tempering allows for the customization of material properties, balancing hardness and toughness according to the needs of specific applications. The process ensures the repeatability and consistency of material properties, crucial for quality control in mass production. In tool steels, tempering improves the ability of tools to develop and sustain a cutting edge by complementing a high degree of hardness with some additional toughness.
Does Tempering Increase Ductility?
Yes, tempering generally does improve ductility in susceptible metals. To learn more, see our guide on Ductile Material.
What Are the Different Types of Tempering?
There are various degrees or types of tempering, tailored to achieve specific mechanical properties in steels, though similar effects and benefits can be delivered in other metals. Listed below are the different types of tempering:
- Full Tempering: The material is heated to a chosen temperature on the tempering scale and then slowly cooled. The temperature of heating defines the relative adjustment of hardness and toughness that results.
- Subcritical Tempering: Performed at lower temperatures. This procedure enhances toughness while maintaining more of the hardness that was previously imposed by heat/quenching.
- Precipitation Tempering: Used for a limited number of alloys. This process involves heating to induce the formation of fine precipitates, generally of one alloying element, to improve strength by altering the crystalline structure with precipitated inclusions, generally of a softer and more ductile constituent.
- Double Tempering: The material undergoes two tempering cycles, further enhancing toughness without excessively sacrificing hardness.
- Low-Temperature Tempering: Performed to retain maximum hardness while moderately improving toughness.
- High-Temperature Tempering: The higher temperature of reheating delivers maximum toughness, at the expense of hardness.
How Does Tempering Work?
At a crystalline level, tempering is a heat treatment process that involves rearranging the internal microstructure of the material, particularly steel, to achieve specific mechanical properties. This discussion assumes that a part has undergone a heat/quench hardening process, which is the stage that tempering generally follows. This heat/quench stage imposes crystalline changes on the material structure, on which tempering serves to alleviate some detrimental effects.
The material is reheated to a lower temperature than that of hardening, typically below the critical point, but generally well above room temperature. This initiates tempering. During tempering, martensite crystals (in steel) undergo recrystallization, reforming into smaller and more stable grains. This reduces internal stresses within the material. The hold or rest time at the tempering temperature can affect the degree of effectiveness. Carbon atoms, which were trapped within the martensite during quenching, start to diffuse within the material. Time must be allowed for the maximization of this stage. This diffusion process allows for the formation of new, less brittle phases, such as ferrite and cementite. The rest time and temperature differential to the surrounding environment allow this recrystallization and diffusion process to complete fully. The final microstructure consists of a mixture of martensite, ferrite, cementite, and other phases. The arrangement and distribution of these phases determine the material's hardness, toughness, and strength.
To learn more, see our article on Steel Microstructure.
Where Is Tempering Commonly Used?
Tempering is used whenever a combination of hardness, toughness, and resistance to fracture is needed to ensure the sharpness, longevity, and stress/shock performance of materials and components.
Tempering is crucial for creating durable and resilient tools, such as: drills, milling cutters, screwdrivers, and saw blades. It is used for high-stress structural, linkage, and engine parts in aerospace applications, in which high strength and resistance to impact are critical. Tempering is employed in the production of engine parts, gears, and suspension components to increase resilience in hardened parts. Structural steel components, such as beams and columns, are tempered to ensure they can withstand heavy and variable tensile, compressive, or mixed loadings under extreme conditions such as earthquakes. Surgical instruments and implant materials require precisely tuned mechanical properties achieved through tempering. Gun barrels, breach blocks, loading, and aiming components are commonly tempered to enhance strength and durability. Critical components used in drilling and extraction operations are tempered for resilience in harsh and downhole environments, in which failures are both costly and time-consuming.
What Are Examples of Tempering Applications?
Examples of tempering applications include:
- Cutting tools like: drills, milling cutters, and lathe tools.
- Automotive parts such as: engine crankshafts, gears, and axles.
- Knives and other kitchen tools.
- Gun barrels.
- Surgical instruments and implant materials.
- Aerospace components such as: aircraft landing gear, engine parts, and structural elements.
- Oil & gas equipment such as: drill bits and wellhead components.
When To Use Tempering?
Tempering is used after the initial quenching or hardening process when a material, typically steel, becomes excessively hard and brittle. It is employed to reduce hardness, improve toughness, relieve internal stresses, and achieve the desired mechanical properties required for specific applications, such as: tools, automotive components, and structural materials.
What Are the Different Stages of the Tempering Process?
The following stages collectively result in the desired balance of hardness, toughness, and other mechanical properties for the tempered material:
1. Heating
The tempering process begins by heating the previously quenched or hardened material to a specific temperature, selected according to: material constituents, state of hardening, and final property requirements. This temperature is typically below the critical point but well above the ambient or normal use environment temperature of the part. The precise temperature depends on the desired mechanical properties and the material being tempered.
2. Dwelling
Once the material reaches the target tempering temperature, it is held or "dwelled" at that temperature for a duration that influences both the crystallization process and the carbon diffusion process which is an active aspect of steel behavior. The dwelling time allows for the internal microstructure to undergo changes, including the diffusion of carbon atoms and the relief of internal stresses.
In precipitation tempering, the dwell time can be more extended, to allow for precipitates to form within the microstructure.
3. Cooling
After the dwell period, the material is slowly cooled in a highly controlled manner. The cooling rate can dramatically affect the final properties of the material. Slow cooling promotes toughness, while rapid cooling can better maintain hardness. The details of the cooling process can be selected depending on the application and material requirements.
How Long Does the Tempering Process Take?
The duration of the tempering process varies depending on several factors, including: the type of material, its thickness, and the desired mechanical properties. Typically, tempering can last anywhere from one to several hours; it is particularly extended by dwell periods that can be very long in some cases. Smaller or thinner components may require shorter tempering times, while larger or thicker parts may need longer periods for the heat to penetrate and the desired microstructural changes to occur.
Tempering times are determined through metallurgical analysis, engineering specifications, and skilled craftsman experience, to achieve the target material properties.
What Are the Metals Used for Tempering?
While tempering is most commonly associated with steel and its alloys, there are other metals and alloys that can undergo similar heat treatment processes to improve their properties. Some of these include: copper alloys, aluminum alloys, nickel alloys, titanium alloys, brass, bronze, and glass.
How Does Carbon Steel React to Tempering?
Tempering of steel significantly improves the metal’s toughness, making it less brittle and more resistant to impact and stress. This is achieved by reducing the internal stresses and refining the microstructure. Carbon steel tempered at specific temperatures achieves a stable microstructure and a beneficial combination of hardness, toughness, and strength. During tempering, carbon steel often exhibits a characteristic color change in the surface oxide film, described as tempering colors. These can be used as a guide to the tempering temperature and therefore the degree of temper applied. These colors range from “straw” through gold, to blue, and then gray, depending on the exposed temperature.
To learn more, see our guide on Carbon Metal.
Can Tempering Be Done to Aluminum?
No, aluminum cannot be tempered in the same way as steel, because it does not undergo a heat/cooling-induced phase transformation similar to that of steel. Aluminum is typically age-hardened or precipitation-hardened to enhance its mechanical properties.
To learn more, see our guide on What is Aluminum Metal.
Can Tempering Be Done to Titanium?
Titanium can be heat-treated, but the process is not referred to as tempering. Instead, titanium undergoes a heat treatment process called annealing or solution heat treatment, followed by aging or precipitation hardening to achieve desired mechanical properties. The end effect of these processes is analogous to the hardening/tempering of steels, however.
To learn more, see our guide on What is Titanium Steel.
At What Temperature Is Tempering Done?
The tempering temperature of metals varies with the material/alloy composition, the degree of temper required, and the desired mechanical properties. However, tempering temperatures for steel and similar alloys generally fall within the range of 300–700 °C.
Low-temperature tempering takes place at 150–350 °C. This results in increased toughness and the retention of relatively high hardness. Medium-temperature tempering occurs at 350–500 °C. This balances hardness and toughness for general-purpose applications. High-temperature tempering takes place at 500–700 °C. This primarily enhances toughness, sacrificing hardness in the process.
How Does the Color of Steel Change When Tempered?
The oxide color of steel changes very predictably with temperature, giving a good approximate indicator of the tempering temperature. For example, at 200–300 °C, steel takes on a pale yellow or straw color. At 300–400 °C, it adopts a gold or brownish-gold color. At 400-500 °C the produced color is gray.
How Many Times Can You Temper Steel?
Steel can be tempered multiple times if necessary, but there are practical limits to the number of tempering cycles a piece of steel can undergo. The number of tempering cycles a steel component can endure depends on several factors: original hardness, alloy, tempering temperature(s), and section thickness. Excessive tempering can eventually lead to a loss of hardness.
To learn more, see our guide on Steel Metal.
Do You Quench After Tempering?
No, you do not quench after tempering. The effective temperament of all appropriate metals requires slow cooling as the final stage. This process allows effective crystal growth.
What Are the Advantages of Tempering?
Tempering offers a range of significant advantages as listed below:
- Reduces the brittleness of hardened materials, enhancing their toughness and resistance to fracture.
- Precise control over the final mechanical properties—various degrees of hardness, strength, and ductility are achievable.
- Helps relieve internal stresses introduced during hardening, reducing the risk of distortion or warping.
- Materials that have been properly tempered are less prone to catastrophic failure and are more resistant to crack propagation.
- Exhibit improved resistance to fatigue and cyclic loading.
- Are often easier to machine, reducing manufacturing costs.
- Ensures the repeatability and consistency of material properties, crucial for quality control in manufacturing.
What Are the Disadvantages of Tempering?
While tempering offers numerous advantages, it also has some potential disadvantages and limitations as listed below:
- Inherently reduces the heat/quench or work hardness of the material.
- Can reduce the wear/abrasion resistance of materials.
- The process can be complex and delicate, requiring precise control of temperature and time. Lack of precision can produce divergence in results.
- The heating process during tempering causes surface discoloration or scaling of the material, requiring additional finishing processes.
- While tempering is versatile, it is not suitable for all materials or applications.
Is Tempering Metal Expensive?
No, the cost of tempering metal is generally not considered high when compared to other heat treatment processes. The degree of temper and the imposition of narrow restrictions on the fine details of the process will increase the cost. However, since it can deliver extreme performance properties, it cannot be considered expensive, given that more extreme applications generally have higher-value outcomes.
How Common Is Tempering?
Tempering of steel is very common and is a very widespread method. It is generally necessary as a post-processing step to tune and optimize the properties delivered by hardening.
What Is the Difference Between Tempering and Annealing?
Tempering and annealing are both processes used to alter the properties of metals, but they serve different purposes and involve distinct procedures. The primary purpose of tempering is to improve the toughness and ductility of a previously hardened material (usually steel or alloys) while retaining a chosen level of hardness. It reduces brittleness and makes the material more suitable for applications requiring a balance between hardness and toughness. Annealing is employed to return materials to their fully softened state, removing all heat/quench or work hardening.
Tempering is used to improve toughness and ductility in hardened materials while maintaining some hardness. Annealing is employed to soften and make materials more workable, reducing hardness significantly.
To learn more, see our article on the Difference Between Annealing and Tempering.
Summary
This article presented tempering, explained it, and discussed its purpose and how it works. To learn more about tempering, contact a Xometry representative.
Xometry provides a wide range of manufacturing capabilities and other value-added services for all of your prototyping and production needs. Visit our website to learn more or to request a free, no-obligation quote.
Disclaimer
The content appearing on this webpage is for informational purposes only. Xometry makes no representation or warranty of any kind, be it expressed or implied, as to the accuracy, completeness, or validity of the information. Any performance parameters, geometric tolerances, specific design features, quality and types of materials, or processes should not be inferred to represent what will be delivered by third-party suppliers or manufacturers through Xometry’s network. Buyers seeking quotes for parts are responsible for defining the specific requirements for those parts. Please refer to our terms and conditions for more information.
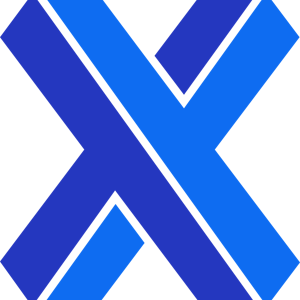