Laser diodes are a diverse family of electrically pumped semiconductor lasers. They differ in operational and construction details and cover a wide range of emission frequencies and powers, but they have many areas of core technology in common. All semiconductor lasers generate light within the active region, the charge depletion zone at the p-n junction of the diode. When a forward-biased electrical current is applied (+ve to the p and -ve to the n) to the diode, “electrons and holes” are driven to the junction regions and they cancel when they meet, releasing a photon in “stimulated emission.” The photons then generally undergo multiple reflections within the diode cavity, amplifying the light through optical gain in a complex, many-bodied interaction between photons, holes, and electrons.
Listed below are the 15 different types of diode lasers:
1. Edge-Emitting Diode Lasers
Edge-emitting diode lasers emit laser light from the edge of the semiconductor chip. They emit light parallel to the chip surface. Edge-emitting diode lasers are formed from a chip made of gallium arsenide (GaAs), indium phosphide (InP), or gallium nitride (GaN). The chip is composed of two (or more) layers, including the charge depletion (active) region at the p-n junction, where the laser effect occurs.
Edge-emitting diodes can provide high optical power levels, ranging from milliwatts to ten watts or more. They also exhibit higher than typical electrical efficiency, compared with most other types of laser and laser diode. These lasers are used in most fields: telecommunications, optical data storage, barcode scanning, laser printing, optical sensing, medical equipment, and industrial laser systems.
2. Vertical-Cavity Surface-Emitting Diode (VCSED) Lasers
VCSED devices are more commonly called vertical-cavity surface-emitting lasers (VCSELs). These are a semiconductor laser diode class that emits laser light perpendicular to the surface of the chip, through the top surface of the chip. VCSELs are formed from a p-n junction chip with a vertical cavity, consisting of two distributed Bragg reflector mirrors. The active region, where light is initiated by charge cancellation, is centered between these mirrors. This laser type generally populates the active region with quantum wells or similar gain-inducing structures. The light is emitted perpendicular to the surface of the chip as a circular or elliptical beam with a well-defined and symmetrical profile. This beam profile responds well to collimation, with relatively low divergence.
These devices have several advantages over edge-emitting diode lasers. Their threshold current is low, allowing high electrical efficiency at low power levels. Those devices with a circular beam profile are well suited to coupling to optical fibers. A major advantage of VCSELs is that they can be manufactured at wafer-scale, resulting in lower production costs and higher uniformity than individually constructed devices.
VCSED lasers are available to emit a range of wavelengths, from mid to near-infrared, and also visible light. The wavelength of output results from material selection, junction design, and resonant cavity form. They are widely used in: fiber-optic networks, optical interconnects, and high-speed data transmission systems. They are also used in 3D sensing for facial recognition and depth sensing in mobile devices, and in more general optical and sensing applications such as optical mice, laser printers, and 3D scanners.
3. Distributed Feedback (DFB) Lasers
DFB (distributed feedback) lasers have a similar structure to other semiconductor lasers. However, the inclusion of a periodic grating structure within the active region, or the external waveguide, is unique to this class. The distributed feedback grating consists of a periodic variation in the refractive index of the waveguide region, resulting in a periodic modulation of the gain profile. This acts as a feedback mechanism, forcing optical feedback/amplification at a selected wavelength while suppressing other modes. This means these devices produce light at a specific wavelength with high spectral purity and narrow linewidth. This is ideal for high-data-rate fiber-optic communications, sensing, and various high-resolution spectroscopy and metrology applications.
DFB lasers can also be designed for wavelength tunability within a limited range. This results from temperature tuning, current tuning, or an external feedback mechanism that allows refractive index adjustment.
4. Quantum Cascade Lasers (QCLs)
The quantum cascade laser (QCL) uses quantum cascade transitions between energy levels within multiple semiconductor junctions as the laser source. QCLs are constructed from multiple quantum wells, with barriers formed by semiconductor layers of various bandgaps. When a forward bias current is applied, electrons and holes travel through multiple quantized energy levels, efficiently generating photons at each transition. They provide emission in the mid-infrared and terahertz regions of the electromagnetic spectrum and can emit a broad range of wavelengths within these regions. The majority of mid-infrared laser technologies need cryogenic cooling, whereas QCLs operate at room temperature, making them ideal for applications needing this frequency range. Delivering high optical power levels makes them suitable for higher-energy applications, delivering continuous-wave (CW) mode with very stable output.
Relatively simple tuning of the emission wavelength is achieved by adjusting layer thicknesses and the bias voltage, making them ideal for spectroscopic analysis applications requiring multiple wavelengths. They are also used for environmental monitoring, medical diagnostic systems, remote sensing, and free-space communication.
5. External Cavity Diode Lasers (ECDLs)
ECDLs are a format of device that utilizes an external cavity, usually an external reflector or grating, to amplify the laser output and control its characteristics. ECDLs allow increased tunability, narrow linewidth, and precise wavelength control compared to other formats of the diode laser. They have a similar structure to other diode lasers, with a forward-biased p-n junction and an active region in which photons are emitted. The external cavity is added to the laser to provide optical feedback that allows for precision tuning of the emission frequency. This cavity houses a reflector, grating, or other optical structure that reflects a proportion of the beam back into the cavity.
ECDLs can facilitate narrower line widths, in comparison to other diode laser types. The external cavity suppresses unwanted longitudinal modes and optical noise, delivering better coherence and a narrower linewidth beam. This class of device is ideal for applications requiring high spectral purity for very precise optical quantization.
ECDLs deliver significantly improved wavelength tunability, in comparison to most diode lasers. The laser wavelength can be precisely tuned by subtly adjusting the position or incident angle of the external reflector or grating. This allows for a wide spectrum of demanding applications in spectroscopy, atomic and molecular physics, and metrology. An advantage of good design in the external resonant cavity is that the adjustment of emission frequency can be mode-hop free—that is, smooth adjustments can be made between desired wavelengths, without asymptotic and disruptive step changes.
6. Tapered Diode Lasers
Tapered diode lasers (or tapered amplifiers; tapered lasers) are a class of lasers with a tapered amplification cavity. These lasers achieve high power output, good beam quality, and high electrical efficiency. This tapered region is wider at the input end and gradually narrows down toward the output end. This tapering serves to increase the beam width and reduce the optical density in the laser amplification region.
The tapered amplification section allows for increased mode area, enabling higher optical power extraction. It also helps to improve beam quality collimation in the output. The taper also increases the efficiency of utilization of the applied pump energy. Another benefit of the taper is to increase the gain spectrum, allowing for a wider range of wavelengths in the output. This tunability is a particularly valuable feature of the class. These devices are widely used in material processing, laser engraving, and laser pumping (for high-power gas and solid-state lasers). Their high power and good beam quality make them suited to demanding applications in which precision, speed, and power are essential.
7. Superluminescent Diode (SLD) Lasers
SLD (superluminescent diode) lasers, also known as amplified spontaneous emission (ASE) sources, are a laser type that merges properties of laser diodes and LEDs. They produce wide-spectrum light of high intensity, rendering them suitable for particular applications in imaging, fiber-optic sensing, and telecoms. SLD lasers generate incoherent light through amplified spontaneous emission. These devices produce a wide bandwidth of light, ranging from tens to hundreds of nanometers, making SLDs suited to applications that require a wide spectral range or high-resolution imaging. SLD delivers very bright output, which is a measure of the optical power per unit solid angle and unit wavelength bandwidth. The high brightness results from amplified spontaneous emission and optical amplification. Their output has a short coherence length compared with conventional lasers. This is the distance over which the electromagnetic waves maintain their phase relationship. This makes them suitable for applications that require low-coherence interference or depth-resolved imaging. SLDs are used in optical coherence tomography (OCT), fiber-optic sensing, spectroscopy, biomedical imaging, optical metrology, and optical testing. They are particularly valuable in OCT systems for high-resolution imaging of biological tissues and materials.
8. Double Heterostructure Lasers
Double heterostructure (DH) lasers are a branch of the laser diode family that integrates a heterostructure that improves the performance of the technology. DH lasers have lower threshold current, higher efficiency, and increased output power, in comparison to the common homojunction construction.
DH lasers are assembled from two p-n junctions formed in three layers. The depletion zone (the center, n-type layer) is coupled between two p-type layers with a wider bandgap. This configuration creates efficient confinement of the carriers and a leak-free optical mode, increasing electrical efficiency and overall performance. Increased carrier confinement helps to increment carrier density and recombination, leading to higher gain and improved operational efficiency in most aspects. A secondary benefit is that the heterostructure induces optical confinement, boosting the light-matter interaction. The lower threshold current results from lower charge-carrier leakage, enabling the laser to reach the threshold for the onset of lasing at lower current levels.
These devices are widely utilized in telecoms, optical data devices, laser printing, and laser-enabled measurement systems. They are particularly valuable in long-distance fiber-optic communications in which high efficiency, low threshold currents, and high output are beneficial.
9. Quantum Well Diode Lasers
Quantum well diode lasers are a family of devices that contain quantum good structures which enhance optical/electrical properties. They achieve lower threshold current, a higher power efficiency, and improved wavelength control, relative to more basic devices. These devices are constructed from a layered structure of thin semiconductor wafers of narrower bandgap, encased in higher bandgap layers. The quantum well layer creates a confinement region for both carriers and generated photons, improving optical amplification. The confined carrier achieves higher density in the quantum well region, which facilitates improved use of carriers for stimulated emission, resulting in improved power conversion efficiency. They allow precise control over the generated wavelength, by adjusting the well width and composition. This allows emission wavelength to be tuned precisely to demanding specifications.
Quantum well diode lasers are renowned for narrow linewidth output. The suppression of longitudinal mode competition and reduced optical noise result in better coherence and narrower spectral behavior. This format of device is particularly beneficial in telecoms, optical data storage, laser printing, and medical diagnostics. Compact and efficient sources of radiation for fiber-optic communications are critical in high bandwidth and long-distance fiber optics.
10. Single Longitudinal Mode Laser (SLM) Lasers
Single longitudinal mode (SLM) lasers emit light to produce a single frequency or wavelength output with high coherence and narrow linewidth. SLM lasers apply various techniques such as mode selection elements, frequency stabilization methods, and cavity design optimization to achieve this single-mode output. Suppression of interfering longitudinal modes generates a highly coherent output with a narrow frequency spectrum.
SLM lasers are used in various fields such as telecoms, fiber-optic sensing, metrology, spectroscopy, and interferometry and as research tools, because of their high coherence, precise wavelength control, and narrow linewidth.
11. Interband Cascade Lasers
Interband cascade lasers (ICLs) operate on the interband transition between different electronic bands within the active region. They deliver efficient and high-performance operation in the mid-infrared wavelength spectrum. ICLs benefit from interband transitions between energy bands within each wafer, exploiting cascaded transitions between multiple stages/quantum wells to achieve enhanced optical gain and laser emission. Conventional diode lasers rely on more limited intraband transitions. They are commonly designed to generate radiation in mid-infrared wavelengths, between 3 to 12 micrometers. Multiple quantum well stages are electrically connected in a cascaded configuration. Each stage participates in the gain process, resulting in higher optical amplification than single junction devices.
ICLs deliver a particularly low threshold current for the onset of lasing. Higher efficiency carrier transport and utilization results in lower power consumption. ICLs are applied to gas sensing, chemical analysis, environmental monitoring, industrial process control, and free-space optical communications. Mid-infrared radiation is useful for detecting and measuring specific pollutants.
12. Separate Confinement Heterostructure Lasers
Separate confinement heterostructure (SCH) lasers use a heterostructure design to improve optical and electrical properties. This delivers reduced optical losses, enhanced carrier confinement, and improved overall performance compared with common homojunction lasers. SCH lasers incorporate several wafers with varying bandgaps to form a more complex heterostructure. The depletion layer is sandwiched by wider bandgap layers. This complexity allows for enhanced confinement of both carriers and optical modes.
Improved confinement and reduced optical leakage result from the cladding layers trapping both optical and charge carrier activity within the active region. Reduced carrier leakage particularly contributes to improved threshold current and electrical efficiency. In turn, this enhances performance, compared to homojunction lasers, by improving temperature stability, higher modulation bandwidth, and temperature-dependent wavelength drift. SCH lasers are particularly useful in applications requiring efficiency and temperature stability. They are suited to general applications like telecoms, optical data storage, laser printing, optical sensing, and laser-based research, but they are particularly suited to harsher environments and fiber-optic communications systems.
13. Distributed Bragg Reflector (DBR) Lasers
Distributed Bragg reflector (DBR) devices are devices that incorporate a distributed Bragg reflector integrated into the amplification cavity. This aspect allows precise control of emitted frequency and narrow filtration for good spectral purity and selection. The Bragg grating consists of alternating layers of high and low refractive index materials that function as a wavelength-selective mirror. This structure reflects light of all non-selected wavelengths while allowing the desired radiation to propagate through the amplification cavity. This structure provides precise wavelength selectivity, and by adjusting the grating period or refractive index pairs, the emitted wavelength can be tuned over a range. This facilitates customization and compatibility with a range of applications, including wavelength-division multiplexing (WDM) systems and optical coherence tomography (OCT).
DBR lasers provide narrow linewidth output as a result of the distributed feedback of the Bragg grating. The grating suppresses undesirable longitudinal modes and results in a single-mode emission of narrow spectral width. These devices deliver beneficial, high side-mode suppression ratios (HSMSR), which represent the power difference between the desired laser mode and the neighboring modes, providing a measure of selectivity, spectral purity, and narrow linewidth.
DBR lasers are used in telecoms, fiber-optic sensing, spectroscopy, metrology, and optical coherence tomography. They are used as precise and stable light sources in various systems that require specific wavelengths, narrow line widths, and high spectral purity.
14. Vertical-External-Cavity Surface-Emitting Lasers
Vertical-external-cavity surface-emitting lasers (VECSELs) are a specialist type of laser device that combines the beneficial features of both vertical-cavity surface-emitting lasers (VCSELs) and external-cavity diode lasers (ECDLs). This results in unique features such as high output power, wavelength tunability, and excellent beam quality.
VECSELs have their laser cavity vertically oriented, so light is emitted perpendicular to the chip surface. This vertical design allows for efficient heat dissipation and precise control over the emitted beam. Their external cavity configuration is constructed from additional reflective surfaces placed outside the chip structure. This enables wavelength control, beam shaping, and power scaling. VECSELs are capable of higher output power than VCSELs because the external cavity configuration improves heat dissipation. Precise wavelength tunability over a broad spectral range is achieved by altering the position of the external cavity mirrors or adjusting the operating temperature of the device. Through precision-designed external cavity use, VECSELs achieve a high-quality output of low divergence angle and uniform beam profile.
VECSELs are used in scientific research, material processing, medical diagnostics, optical sensing, and telecoms. They serve precision applications like laser spectroscopy, laser cooling and trapping/manipulation of atoms, laser ablation, and high-data-rate optical communications.
15. Multi-Longitudinal Mode (MLM) Lasers
Multi-longitudinal mode (MLM) lasers offer the unusual facility of emitting on several, closely spaced but narrow frequency bands, across a relatively broad spectrum. The longitudinal modes in MLM lasers are narrowly spaced. The spacing depends on the functional design of the resonant cavity such as its length and the refractive index of the laser medium. Their broad emission spectrum is due to the presence of these multiple modes. The spectral width and distribution of the modes arise because of cavity and junction design and also from the operating conditions.
MLM lasers are used in spectroscopy, metrology, interferometry, and telecoms. They are particularly applicable to optical coherence tomography (OCT), in which high-resolution imaging is possible, resulting from the interference of the multiple longitudinal modes.
What Is a Diode Laser?
A diode laser is a semiconductor device that emits coherent and generally narrow monochromatic light through the process of stimulated emission. Its operational principle involves the emission of a photon for each charge cancellation in the depletion region at a p-n junction. Some varieties of the diode laser emit light in different arrangements such as: multiple monochromatic bands across a wide spectrum, or wider band polychromatic light. They vary in beam forming, beam quality, coherence, and linewidth, due to a range of design formats and material choices.
For more information, see our How Does a Laser Diode Work guide.
How Does a Diode Laser Work?
In the simplest type, a single forward biased p-n junction experiences interactions of holes and charges driven by the bias voltage. At each hole/charge meeting, a coherent and specific-frequency photon is emitted. Photons are usually partially trapped within a semi-reflective cavity that allows them to repeat interactions with the semiconductor material and/or charges within it, resulting in additional stimulated emission and enhancing beam strength
How Important Are Diode Lasers?
Diode lasers have a huge range of medical, scientific, industrial, and consumer-product applications, and they are a cornerstone component family within a surprising range of technologies. This results from their: versatility, compact size, efficiency, direct current operation, wavelength range, cost-effectiveness, and reliability. The importance of diode lasers is evident from their widespread adoption across industries and the broad range of applications they serve.
What Is the Primary Use of a Diode Laser?
The numerically most important use of diode lasers is optical data transmission in telecoms. Diode lasers are key to fiber-optic communication systems to transmit data over long distances with high bandwidth. This results from their best-in-class functions such as:
- High-data-rate capability
- Compact package size
- Electrical efficiency
- Wavelength compatibility
- Direct modulation capability
- Long operational life span/reliability
What Are Some Applications of Laser Diodes?
Laser diodes are heavily employed across many fields, including:
- Printing
- Engraving and marking
- Cutting
- Fiber-optical communication
- Medical and aesthetic treatments
- Optical data storage
- Barcode scanning
- Sensing and metrology
- Display and projection of images
- Spectroscopy and chromatography
- Scientific research
How To Choose the Type of Laser for Your Need?
Choosing the right type of laser for your specific needs requires considering several key factors such as:
- Application: Understanding your application requirements will help narrow down the suitable laser types.
- Output Power and Input Energy Requirements: Some applications may require high-power lasers for tasks like cutting thick materials, while others may require low-power lasers for precision tasks such as engraving, and still others may require electrical power efficiency for portable applications.
- Wavelength: The choice of wavelength depends on the details of the application such as the material to be processed, absorption characteristics, data rate, transmission characteristics, etc.
- Beam Quality: Some applications may require high-quality and excellent collimation, whereas others may be less sensitive to beam quality than device cost.
- Reliability and Maintenance: If low maintenance is a requirement, diode laser sources are ideal—but there are differences across the class that might influence selection.
- Cost: Where cost is a determining factor, device simplicity is usually beneficial. Remember cost is not just device cost, but long-term ownership cost, which may alter the selection.
How Can Diode Lasers Be Used in Laser Cutting?
Diode lasers are well-suited to cutting applications that involve thin materials and relatively low-power cutting requirements. The use of diode lasers of all types is increasing in cutting applications. Frequency selection, linewidth, beam quality (of some laser diode types), power efficiency, compact size, and particularly device life/maintenance benefits are critical factors in this. For more information, see our How Does Laser Cutting Work guide.
Can Diode Lasers Be Used for Laser Welding?
Yes, diode lasers are suited to some laser welding applications, particularly where their characteristics align with the requirements of the process. These are some advantages diode lasers offer in welding applications:
- Diode lasers have fast modulation and switching capabilities. This provides for rapid on/off switching for tight control and rapid processing of complex welds.
- Deep penetration is possible in certain materials. Closely focusing the beam and using appropriate power settings can generate high specific energy at the melting point that can penetrate deep into the material with a limited heat-affected zone.
- Joining dissimilar materials by providing a concentrated and localized heat source allows for controlled and precise distribution of heat into materials with different thermal characteristics.
- Compact welding devices result from high energy density in the laser and limited need for ancillary devices such as cooling systems.
- They are cost-effective compared with other laser types like fiber lasers or solid-state lasers.
Is It Possible To Use Diode Lasers for Engraving?
Yes, diode lasers can be used for engraving, particularly for applications that require precision and low-power engraving. While diode lasers may not be as commonly used as fiber lasers or CO2 lasers for industrial engraving on a wide range of materials, they can still be effective in certain scenarios.
Summary
This article presented 15 different types of diode lasers, explained them, and discussed how each are used. To learn more about diode lasers, contact a Xometry representative.
Xometry provides a wide range of manufacturing capabilities, including sheet cutting and other value-added services for all of your prototyping and production needs. Visit our website to learn more or to request a free, no-obligation quote.
Disclaimer
The content appearing on this webpage is for informational purposes only. Xometry makes no representation or warranty of any kind, be it expressed or implied, as to the accuracy, completeness, or validity of the information. Any performance parameters, geometric tolerances, specific design features, quality and types of materials, or processes should not be inferred to represent what will be delivered by third-party suppliers or manufacturers through Xometry’s network. Buyers seeking quotes for parts are responsible for defining the specific requirements for those parts. Please refer to our terms and conditions for more information.
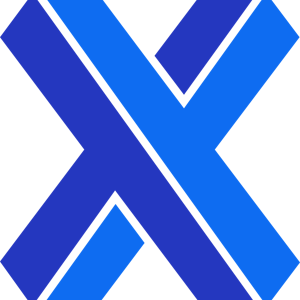