Shear stress is a fundamental concept in mechanics and materials science. It is a pivotal idea to understand in terms of how forces act within solids, fluids, and structures. This critical parameter quantifies the internal forces that arise when adjacent layers of a material or fluid slide or deform relative to each other.
This article will discuss the key aspects of shear stress, exploring its definition, causes, significance in engineering, practical applications, and implications.
What Is Shear Stress?
Shear stress is a fundamental concept in physics and engineering that describes a material’s internal resistance to deformation when subjected to a force or load that acts parallel to your selected plane of reference. It is a measure of how easily one plane within the material can be made to slide or deform along another in response to an applied force. In practical terms, when you apply a shear force to a solid object, it deforms the object's shape, causing different layers of the material to slide past each other. The rate at which this deformation occurs depends on the material's shear modulus, which is a measure of its resistance to shear deformation.
What Is the Another Term for Shear Stress?
Another term for shear stress is tangential stress.
Is Shear Stress and Shear Force the Same Thing?
No, shear stress and shear force are related but they are not equivalent. Shear force is an internal force generated by an applied load. It is typically depicted in shear diagrams for different sections along a structural member and appears as a pair of vector forces of equal magnitude but opposite directions.
Shear stress, on the other hand, is a measure of the intensity of this force distributed over a unit area. It is expressed in units of force per unit area (e.g., Pascals or N/m²). Shear stress is defined as a type of stress that acts coplanar with a given cross-section of the material. It arises from shear forces. These forces can cause deformation or sliding of material layers relative to each other, and shear stress quantifies how easily this deformation occurs.
Is Shear Stress the Same as Yield Strength?
No, shear stress and yield strength are distinct but related mechanical properties. Both characterize how materials respond to external forces. Shear stress measures the internal resistance of a material to deformation when subjected to a force acting parallel to its surface and is expressed in units like Pascals or N/m².
On the other hand, yield strength denotes the point at which a material begins to undergo plastic deformation when subjected to an applied load or stress and is usually measured in stress units such as pascals or megapascals (MPa). When a material exceeds its yield strength, it experiences permanent deformation. Although these properties are distinct, they are related in the sense that a material's yield strength dictates the maximum shear stress it can endure before permanently deforming.
To learn more, see our guide on Material Yield Strength.
How Does a Shear Stress Work?
Shear stress occurs when the material or fluid’s adjacent layers move relative to each other due to an applied force. This results in a component of the force per unit area acting parallel to the surface. This force attempts to deform the material by causing one portion of it to slide past another. It is typically represented by the symbol "τ" (tau). Shear stress is measured in units of force per unit area, such as pascals (Pa) or newtons per square meter (N/m²). To calculate shear stress, divide the magnitude of the applied shear force by the area over which it acts.
Two primary directions are listed for shear stress: positive and negative. Positive shear stress arises when an applied force induces deformation by causing one part of a material to slide relative to another part along the positive x or y-axis.
How Important Is Shear Stress?
Shear stress holds immense importance because it helps explain material and fluid behavior. Its significance becomes evident in structural engineering, where it's crucial for designing and assessing the stability and load-bearing capacity of structures like buildings, bridges, and aircraft. In fluid dynamics, shear stress is indispensable, aiding in the understanding of fluid behavior, viscosity, and pressure distribution, thereby guiding the design of pipelines and hydraulic systems. Material science benefits from shear stress analysis, especially in investigating how materials deform or fail under different loads, impacting the use of metals, polymers, and composites.
What Is the Cause of Shear Stress?
Shear stress is the result of external forces or relative motion that prompts one layer of a material or fluid to move or deform parallel to an adjacent layer. Its origins vary depending on the context and the substance involved. In structural materials like beams, shear stress emerges from mechanical forces applied parallel to the material's surface, often due to external loads. In fluid dynamics, it arises as a consequence of fluid layers moving at different velocities or when a fluid flows over a solid surface. This is described by Newton's law of viscosity. Geologically, tectonic forces generate shear stress when rocks follow fault lines to slide past each other, potentially leading to seismic activity.
Is Shear Stress a Pressure?
No. Even though it uses the same units, shear stress cannot be interpreted as pressure. Pressure is a form of normal stress, meaning it acts inward, toward a surface, and perpendicular to that surface. Essentially, it exerts a uniform force distribution that compresses or affects the material in all directions away from the compressed surface. In contrast, shear stress is tangential stress, acting along the surface and parallel to it. Shear stress induces relative motion or deformation in adjacent layers within a material or fluid. Furthermore, while stress is internal and can result from external forces, pressure typically only refers to forces applied externally to a surface or object.
What Are the Signs of Shear Stress?
Evidence of shear stress can take different forms based on: the magnitude of the shear stress, the material's properties, and the loading conditions. In ductile materials like metals, shear stress can result in plastic deformation, which may not lead to immediate failure. However, if the shear stress exceeds the material's yield strength, it can cause permanent deformation and, over time, contribute to failure. Conversely, brittle materials like ceramics and certain types of glass are more prone to shear-induced failure, as this mode can initiate cracks or fractures that propagate quickly through the material. This phenomenon is particularly relevant in structural engineering, where excessive shear stress can lead to shear failure in components such as beams and columns.
What Is the Formula for Shear Stress?
Shear stress can be described by different formulas in different scenarios. First, let’s consider the general shear stress equation:
τ = F / A
Where:
τ = shear stress (in Pa or N/m²)
F = force applied parallel to the surface (N)
A = cross-sectional area of the material through which the force is applied (m2)
For beam shear (internal stress within a beam that arises due to the application of a shear force on the beam):
τ = VQ/It
Where:
V = internal shear force within the beam, (N)
Q = static moment of an area (mm²) or m²)
t = thickness of the area of interest (m or mm)
I = the moment of inertia (mm^4)
Finally, shear stress in fluids (liquids and gasses) relates it to the velocity gradient within the fluid:
τ = μ (du/dy)
Where:
τ (tau) = the shear stress in the fluid (Pa)
μ (mu) = dynamic viscosity of the fluid (Pa·s or poise [P])
(du/dy) = velocity gradient, also denoted as ∂u/∂y, where "u" represents the velocity component parallel to the direction of flow, and "y" represents the perpendicular distance.
How Do You Calculate Shear Stress?
To calculate shear stress, you'll need to determine the force acting parallel to a specific surface and the area over which this force is distributed. The basic formula for shear stress is
τ = F / A,
where τ represents shear stress, F is the applied force, and A is the area involved.
First, identify the force acting in parallel to the surface of interest, whether it's due to mechanical loads, fluid flow, or other factors. Next, establish the area perpendicular to the force's direction. Depending on the shape, you can calculate the area using relevant geometry formulas or directly measure it. After obtaining both values, plug them into the shear stress formula, making sure you use consistent units for force and area. The result will be expressed in units of force per unit area, often measured in pascals (Pa) in the SI system. Remember that shear stress is a vector, so its direction, parallel to the surface, is integral to a complete description of the stress state.
The calculation method can vary depending on whether you're dealing with fluids, solids, or specific well-studied materials, so it's crucial to consider the context. The respective equations can be used for beam shear or shear stress in fluids.
How to Calculate Shear Stress in Beam?
Shear stress in a beam can be calculated using the beam shear equation:
τ = VQ/It
Where:
V = internal shear force within the beam
Q = static moment of area
t = thickness of the area of interest
I = the moment of inertia
What Does a High Shear Stress Mean?
A high shear stress indicates that a significant force is acting parallel to a surface per unit area. The interpretation of high shear stress can vary depending on the context in which it occurs. In fluid dynamics, high shear stress may signify resistance to flow or rapid deformation within a fluid, as seen in turbulent flows or fluids flowing across solid surfaces. In structural engineering, it suggests that materials are experiencing substantial forces that may lead to deformation or shear.
What Is the Average Shear Stress?
The average shear stress acting on the unit surface contact area is the cumulative effect of shearing forces generated by all the contacting grits within this specific unit area. Shear stress is determined by dividing the shear force by the object’s cross-sectional area. Similarly, the average shear stress is computed by dividing the shear force by the average cross-sectional area.
What Is an Example of Shear Stress?
Shear stress occurs in many everyday activities and scenarios. Here are some real-life examples:
- When you cut fruits, vegetables, paper, or cloth, the blade exerts shear stress to slide through the material.
- Shear stress is present when your feet push against the ground to move forward.
- When a vehicle accelerates, decelerates, or turns, shear stress acts upon various components like the seats.
- In rivers, water flow applies shear stress to the riverbed, which can lead to erosion and changes in the river's landscape.
What Is the Shear Stress of Metal?
It depends. Every metal can withstand different amounts of shear stress. The specific amount on a given metal object depends on the type of metal, its composition, its shape, and the environmental conditions it encounters. Different metals, such as: steel, aluminum, copper, and titanium, exhibit distinct shear stress characteristics. This property is often determined through laboratory testing and is expressed in units of pascals (Pa) or similar pressure units. The composition of the metal, including any alloying elements, can significantly influence its shear strength. Additionally, factors like temperature, strain rate, and the metal's microstructure — including grain size and defects — can all play roles in its shear stress behavior.
To learn more, see our Metalloids: Properties and Uses guide.
What Is the Shear Stress of Steel?
It depends. The shear stress of steel can vary depending on the specific type of steel, its composition, and other factors like the specific grade and treatment. To learn more, see our guide on Steel Properties.
The shear strength of different steels are given in Table 1 below:
Metal Type | Shear Strength (N/mm^2) |
---|---|
Metal Type Low Carbon HR Steel | Shear Strength (N/mm^2) 345 |
Metal Type Low Carbon C.R. Sheet | Shear Strength (N/mm^2) 276 |
Metal Type ASTM A-36 | Shear Strength (N/mm^2) - |
Metal Type 45-50 Carbon HR Sheet | Shear Strength (N/mm^2) 552 |
Metal Type Spring Steel 1074, 1095 Hardened to Spring Temper | Shear Strength (N/mm^2) 1,380 |
Metal Type COR-TEN Steel | Shear Strength (N/mm^2) 379 |
Table Credit: https://unipunch.com/support/charts/material-specifications/
What Is the Shear Stress of Plastic?
It depends. Shear stress quantifies the internal tension that emerges as molecules within the plastic material slide and exerts forces on one another due to their relative motion. Excessive stress can lead intermolecular bonds to break. Typically, the maximum shear stress a material can endure is approximated to be around 1% of its tensile strength.
What Are the Advantages of Shear Stress?
Listed below are some of the advantages of understanding shear stress:
- Shear stress analysis helps engineers determine the stability and load-bearing capacity of structures like bridges, buildings, and aircraft.
- Shear stress is used in material science to characterize the behavior of materials under shear forces.
- This is a key parameter in fluid dynamics that helps describe the behavior of fluids, including viscosity and flow patterns.
- Industries intentionally introduce shear stress in processes such as mixing, stirring, and cutting. This facilitates the mixing of materials, emulsification, and chemical reactions.
- Known values are used in laboratory settings to evaluate the properties of materials, assess their behavior under various loads, and determine their suitability for specific applications.
What Are the Disadvantages of Shear Stress?
Here are some of the disadvantages and potential issues associated with shear stress:
- Excessive shear stress can lead to structural failure. In solids and structures, shear stress can cause deformation, cracking, or shearing along planes.
- Repeated or cyclic shear stresses can induce material fatigue, leading to the gradual degradation of materials over time.
- The same forces that generate shear stress cause wear and tear on moving parts and surfaces in machinery and equipment. Over time, this can result in the need for maintenance and replacement of components.
- In fluid dynamics, high shear stress can lead to turbulence, which can increase energy consumption and affect the efficiency of fluid systems. It can erode and damage pipelines and equipment.
- In aerospace engineering, aircraft and spacecraft structures must be carefully designed to withstand shear-induced aerodynamic forces.
- Manufacturing processes often need to control and optimize shear stresses to accomplish their goals. Achieving the desired shear effects, such as mixing or cutting, can require careful design and monitoring of equipment.
- High shear stress can pose safety concerns in various contexts, such as vehicle accidents, where abrupt deceleration or impact forces place significant shear stresses on components and occupants.
What Is the Difference Between Shear Stress and Stress?
Shear stress represents a subset of stress in general. Stress is a broader term that encompasses various types of internal resistance within a material that’s subjected to external forces. It's typically represented as a force per unit area and can occur in different ways, including tensile stress (stretching), compressive stress (compression), and shear stress (shearing).
Shear stress, on the other hand, is a specific type of stress that arises when external forces act parallel to the object’s surface or a select cross-sectional plane, attempting to cause one layer of the material to slide past an adjacent layer. It is a form of stress specifically associated with deformation due to shearing or sliding forces.
To learn more, see our guide on Types of Stresses.
What Is the Difference Between Shear Stress and Bending Stress?
Shear stress arises from forces that act either parallel to the material’s surface or act in a way that would cleave the object and slide the cleaved surfaces across one another. It aims to induce one part of the material to slide past another, leading to shearing deformation. In contrast, bending stress results from forces that cause the material to bend or flex as shown in Figure 1:
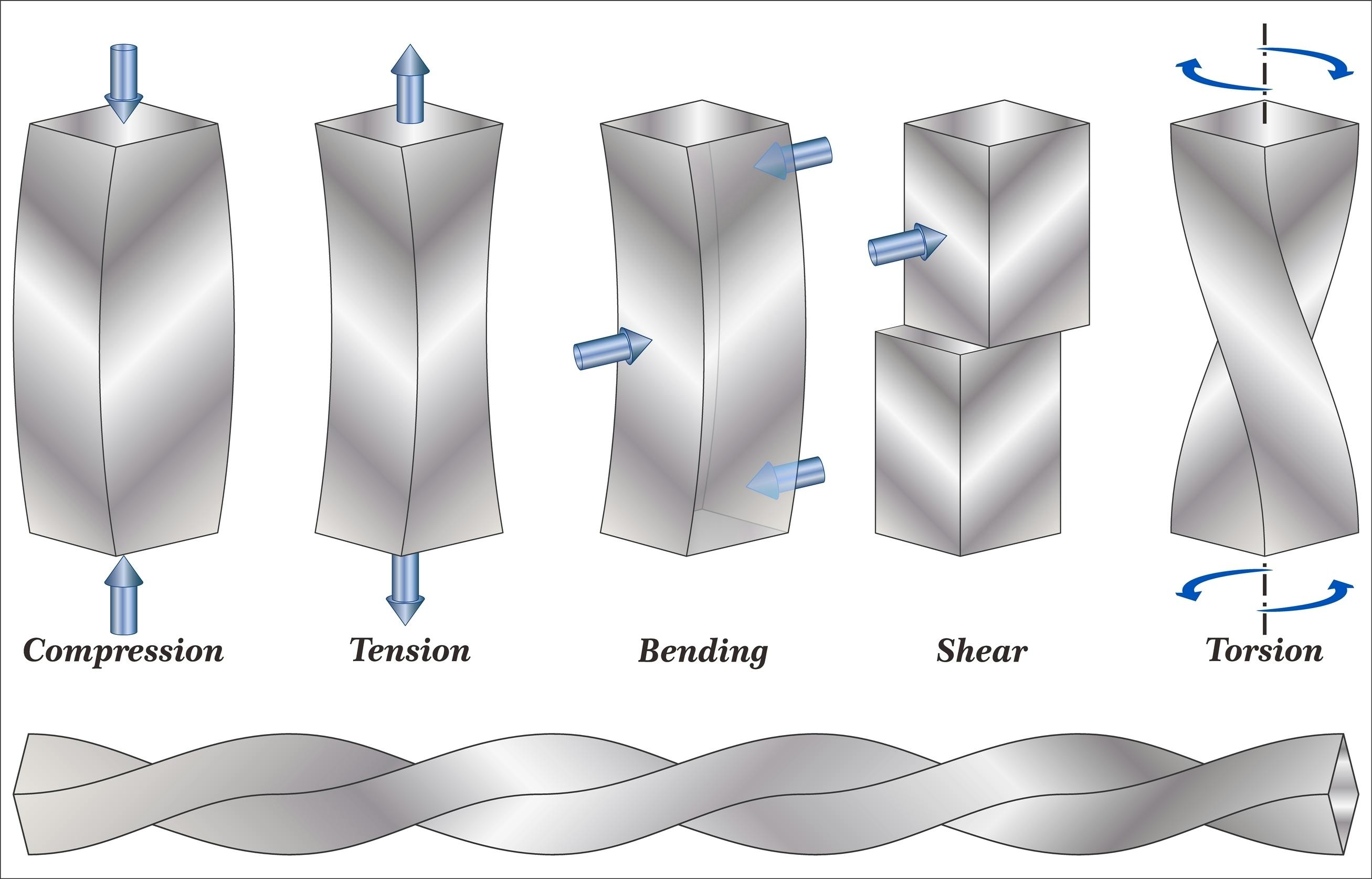
Different types of stresses.
Image Credit: Shutterstock.com/Fouad A. Saad
Shear stress is distributed uniformly across the material's cross-section, while bending stress varies, peaking at the outermost fibers and diminishing towards the center. The equations for calculating them are also different, with shear stress expressed as τ = F / A and bending stress as σ = Mc / I, where F represents the applied force, A is the cross-sectional area, M is the bending moment, c is the distance to the outer fibers, and I is the moment of inertia.
Summary
This article presented shear stress, explained it, and discussed how it works and its advantages. To learn more about shear stress, contact a Xometry representative.
Xometry provides a wide range of manufacturing capabilities and other value-added services for all of your prototyping and production needs. Visit our website to learn more or to request a free, no-obligation quote.
Disclaimer
The content appearing on this webpage is for informational purposes only. Xometry makes no representation or warranty of any kind, be it expressed or implied, as to the accuracy, completeness, or validity of the information. Any performance parameters, geometric tolerances, specific design features, quality and types of materials, or processes should not be inferred to represent what will be delivered by third-party suppliers or manufacturers through Xometry’s network. Buyers seeking quotes for parts are responsible for defining the specific requirements for those parts. Please refer to our terms and conditions for more information.
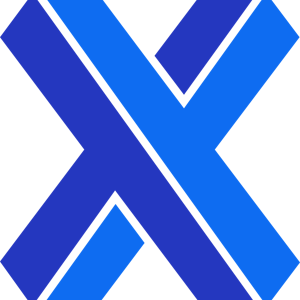