Elastic modulus, also known as the modulus of elasticity, is a significant mechanical property that describes a material’s stiffness. Calculating a material’s elastic modulus helps engineers determine the material’s ability to retain its shape when a load is applied and is fundamental to designing safe structures. There are four types of elastic modulus. Each is associated with a different type of deformation. It is important for engineers to refer to the correct elastic modulus value when designing parts or structures for a particular application.
Elastic modulus can be determined mathematically from Hooke’s law, which describes the relationship between force and the displacement experienced by a spring. Elastic modulus can be thought of as a form of Hooke’s Law since elastic materials act similarly to springs in the elastic region of the stress-strain curve. Like stress, the modulus of elasticity is reported as a force per unit area and is often shown in gigapascals (GPa) or pounds per square inch (psi). This article will review the five main steps in calculating elastic modulus and provide a brief overview of its significance and meaning.
1. Choose a Testing Method
The first step in calculating the elastic modulus is to determine what type of elastic modulus information is needed for the particular application. Each modulus gives insight into how a material behaves when subjected to a different type of loading. Young’s modulus describes elasticity under tensile and compressive loads. Shear modulus, on the other hand, describes elasticity in conditions of shear stress. Bulk modulus refers to elasticity in all directions. Finally, flexural modulus is related to elasticity in bending.
The ASTM standards A-370, E-804, D-638, and D-3039 describe the necessary geometry for different test specimens depending on the test specimen material and the specific modulus being tested for. The test procedure for determining the modulus of elasticity is essentially the same procedure for determining the strength of a material. The appropriate procedure for the desired stress conditions (tensile, compressive, shear, flexural, hydrostatic) should be followed. These procedures are standardized and can also be found in the mentioned standards.
2. Perform the Experiment
Once the test specimen is prepared, a universal testing machine or materials testing system is used. This machine automatically and continuously records the increasing load on the sample and the resulting change (deformation) in the gauge section. This information is converted to stress and strain, and this stress and strain information is used to calculate elastic modulus.
3. Calculate Stress
Many materials testing systems will automatically graph the stress versus the strain during a strength test. Stress is easily determined from the stress-strain curve. If calculating stress without a stress-strain curve, apply a known load to a test specimen and divide the magnitude of the load by the cross-sectional area of the material where the load is applied. For more information, see our guide on Material Stress.
4. Calculate Strain
As with stress, many materials testing systems will automatically graph strain on the X-axis of a stress-strain curve. From the curve, strain is easily determined. However, if a stress-strain curve is not used, strain is determined by applying a known load to a test specimen and determining the deformation of the part when the load is applied. Strain is defined as the ratio of the change in length of a material to its original length. Finally, determine strain by dividing the change in specimen gauge length by the original length. For more information, see our guide on How to Calculate Strain.
5. Calculate the Elastic Modulus
The elastic modulus is the slope of the straight-line elastic (recoverable strain) part of the stress-strain curve traced out by the testing machine. Modern equipment will determine the slope and report it automatically. If the modulus has to be calculated, the simplest method is to simply select two points that are on the linear part of the slope and calculate the slope (rise-over-run) from stress divided by strain. It is customary to use a statistical method such as least-squares fit to make sure that only the straight part of the stress-strain curve is used in the calculation. Elastic modulus has the same units as stress since strain is a unitless value. Once the elastic modulus is obtained, engineers have more insight into how the material will behave when different types of loads are applied.
What Is Elastic Modulus?
Elastic modulus is a number that characterizes a material’s elasticity and its ability to resist elastic, non-permanent deformation. There are four different types of elastic modulus: Young’s modulus, shear modulus, bulk modulus, and flexural modulus. Each type of modulus characterizes a material’s stiffness with respect to particular conditions of applied stress. Higher elastic modulus values mean that a material is more rigid or stiff, while lower elastic modulus values mean that a material is more flexible and malleable.
Elastic modulus can be computed algebraically through Hooke’s law or determined graphically from a stress-strain curve. From Hooke’s law, the force experienced by a spring is equal to its displacement and spring constant. Similarly, Hooke’s Law can be applied to elastic materials where the stress experienced by an elastic material is equal to the material’s stiffness (modulus of elasticity) and strain (displacement). Solving Hooke’s Law equation for the elastic modulus shows that the elastic modulus is equal to the ratio of stress to strain. If the applied stress and strain are known, the modulus of elasticity can be easily determined. On a stress-strain curve, the modulus of elasticity is the slope of the linear portion of the graph, where stress and strain are directly proportional to each other. For more information, see our guide on What is an Elastic Modulus?
What Is the Importance of Calculating Elastic Modulus?
Elastic modulus describes how stiff a material is. Knowing a material’s stiffness, along with knowledge of the applied stress at which deformation will switch from recoverable (elastic) to permanent (plastic) enables one to calculate how much stress can be applied without permanent damage to a structure or component. To accommodate the calculated stresses, a material with a high enough modulus should be selected. Otherwise, the geometry of the part (larger x-section, for example) can be changed to accommodate the anticipated stress while experiencing only elastic deformation. Limiting deformation to the elastic region will improve a material’s durability and provide a safety margin of performance to avoid the risk of catastrophic failure and potential harm to people and property.
What Is the Formula for Elastic Modulus?
The formula for elastic modulus is derived from Hooke’s law. The formula for elastic modulus is a type of Hooke’s law where stress, elastic modulus, and strain replace force, spring constant, and displacement respectively. By algebraically solving for elastic modulus, it can be shown that elastic modulus is equal to the ratio of stress-to-strain. The formula for elastic modulus as derived from Hooke’s law is shown below:
E=𝝈/ε
Where:
E= Elastic modulus (Pa or psi)
𝝈 = stress (Pa or psi)
ε = strain (unitless)
The equation above is for tensile/compressive modulus. Stress (𝝈) is the force per unit area applied perpendicularly to the cross-section of interest, and ε is the change in length along the direction of applied stress. These quantities are the stress and strain experienced in a uniaxial tensile test. If testing for other types of modulus, 𝝈, and ε are replaced by the appropriate type of stress and strain respectively. For example, to calculate shear modulus, the shear stress and shear strain are used. Table 1 below shows the symbols that pertain to each variable for each type of modulus.
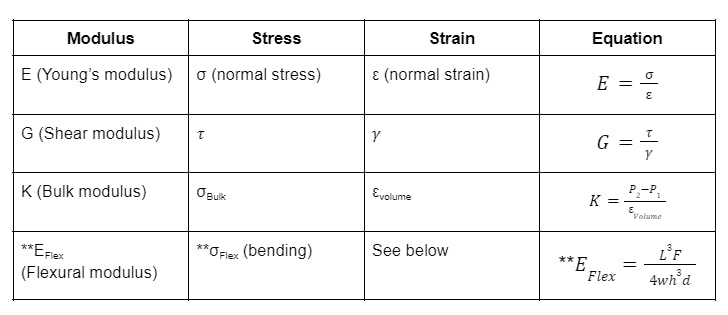
Table 1: Symbols and Equation for Each Type of Modulus
The flexural modulus equation differs from the equations for the other modulus types due to the elastic beam theory.
What Are the Different Types of Elastic Moduli?
There are four different elastic modulus values. Each describes the behavior of a material under different loading conditions. The four types of elastic moduli are listed and described below:
- Young’s Modulus: Young’s modulus describes the ability of a material to withstand deformation when subjected to uniaxial tensile or compressive loads.
- Shear Modulus: Shear modulus describes the ability of a material to resist shear deformation. Shear deformation occurs when shear loads (loads parallel to the cross-sectional area) are applied to a material.
- Bulk Modulus: Bulk modulus characterizes a material’s ability to withstand deformation when subjected to triaxial or isostatic loads.
- Flexural Modulus: Flexural modulus characterizes a material’s ability to withstand deformation due to bending loads.
How Are the Values of Elastic Modulus Expressed?
Elastic modulus is expressed in the same units as stress or applied force per unit area. Common units for elastic modulus are MPa or GPa in SI units, and psi or ksi in English/Imperial units.
What Material Has the Highest Elastic Modulus?
Diamond has the highest elastic modulus. The modulus of elasticity, specifically Young’s modulus, of a diamond is approximately 1220 GPa. This is more than double the elastic modulus of osmium, the metal with the highest elastic modulus.
What Does a Large Elastic Modulus Value Indicate?
A large elastic modulus denotes that a material is highly resistant to elastic deformation and therefore highly rigid. For a given level of applied stress, a material with a high elastic modulus will deform less than a material with a low modulus. Consider a material that is subjected to a stress of 100 MPa. If the material’s elastic modulus is 10 GPa, the applied stress will result in a strain (elastic deformation) of 0.01, whereas if the modulus is 100 GPA, the strain will only be 0.001. The higher value of modulus results in less deformation.
What Does a Small Elastic Modulus Value Indicate?
A smaller elastic modulus denotes that a material is more susceptible to elastic deformation and therefore more flexible. Consider a material where a stress of 100 MPa is applied. If the material’s elastic modulus is 10 GPa, the applied stress will result in a strain (elastic deformation) of 0.01, whereas if the modulus is 1 GPA, the strain will be 0.1. The lower value of modulus results in more deformation.
What Is the Elastic Modulus Symbol?
The capital letter “E” is often used to denote a material’s elastic modulus and refers to Young’s modulus. The capital letter “G” refers to the shear modulus, while the capital letter “K” refers to the bulk modulus. EFlex refers to the flexural modulus.
Are Stress and Strain Both Necessary for Calculation of Elastic Modulus?
Yes, both stress and strain are necessary for the determination of elastic modulus. This is because each type of elastic modulus value is defined as the ratio of stress to a strain given a particular set of loading conditions. For more information, see our guide on the Material Stress-Strain Curve.
What Is the Difference Between Elastic Modulus and Shear Modulus?
Elastic modulus is a general term that refers to a material’s ability to withstand elastic deformation. If not otherwise specified, elastic modulus refers to a material’s ability to withstand elastic deformation when uniaxial tensile or compressive loads are applied. Shear modulus is a type of elastic modulus that specifically quantifies a material’s ability to resist shear deformation. Shear deformation occurs in a direction parallel to the cross-sectional area at which shear stress is applied. For more information, see our guide on What is Shear's Modulus?
Summary
This article presented copper, explained it, and discussed its various applications. To learn more about copper, contact a Xometry representative.
Xometry provides a wide range of manufacturing capabilities, including casting and other value-added services for all of your prototyping and production needs. Visit our website to learn more or to request a free, no-obligation quote.
Disclaimer
The content appearing on this webpage is for informational purposes only. Xometry makes no representation or warranty of any kind, be it expressed or implied, as to the accuracy, completeness, or validity of the information. Any performance parameters, geometric tolerances, specific design features, quality and types of materials, or processes should not be inferred to represent what will be delivered by third-party suppliers or manufacturers through Xometry’s network. Buyers seeking quotes for parts are responsible for defining the specific requirements for those parts. Please refer to our terms and conditions for more information.
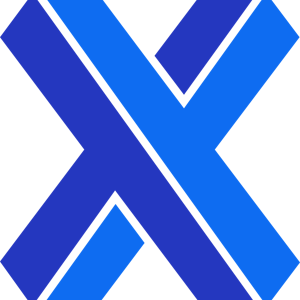